Post-Scarcity Trends, Capacity and Efficiency
Evaluating Design
Examining the surface of Earth today, a network layer of communities, industrial centers, transport routes, recreational areas, agricultural systems and the like dominate much of the landscape. Whether intended as a total system construct or not, this result, at any given point in time, constitutes the appearance of a topographical design.
Yet, on the other hand, given that this resulting “design” today is, actually, a consequential amalgamation of mostly business dynamics - moving money around for personal or group self-interest, based around decision-making mechanisms such as profit, cost-efficiency and the prevailing logic surrounding property relationships – it could also be argued that what has manifest is actually not a “design” at all. Rather, it is rooted in a mechanism that has created the appearance of design ex post facto[2] since the structural outcome recognized was not fully anticipated as a whole prior to its construction.
In other words, the technical order we see in the world today is mostly the result of financial processes that have little to no perception of larger scale structural outcomes. It is more of a proxy system[3] and while there are some relative exceptions, such as the placement of highways, pipelines and the like by funded city planners who simply must take a broad physical view to be functional, even those circumstances are often working around pre-existing property claims and other forms of interference which tend to reduce design efficiency on the whole.
This is an interesting observation, as once it is recognized that our society operates without a large scale preconception of its own physical design, one might begin to realize the enormous level of unnecessary waste and technical inefficiency inherent to such a short-sighted process.
To consider this more so, two points are worth considering:
a) Existing yet Unapplied Solutions
b) Broad Conception vs. Spontaneous Conception
a) Existing yet Unapplied Solutions
This first point concerns the tendency of many new innovations for problem resolution to go unapplied within the current economic tradition.[4] If further life improving methods or technologies have not found their way into a system within a respectable amount of time (or at all) after general validation, we can rightly assume there are inefficiencies, if not deficiencies, with the very process of economic incorporation and development.
In other words, this delay between proven solutions, and their application in the real world, gauges the ability of the socioeconomic system to adapt properly to improved methods and applications. If, for some reason, the social order in question is not able to incorporate such new means to further ecological balance, improve public health, solve problems and increase prosperity, then there is likely a structural problem inherent.[5]
b) Broad Conception vs. Spontaneous Conception
Secondly, from a strictly formulaic viewpoint, direct, total system considerations will always be more efficient and effective than “spontaneous” generation by processes blind to the final outcome or purpose.[6]
In other words, as gestured before, a basic good, such as a car, has a design that is conceived of in advance, before physical production. Once this design is decided upon, it is then followed by applying real life materials and processes to create the actual physical product. This may seem obvious to most as a logical process but the relevance of such preconception is often lost when it comes to larger order contexts.
We have to wonder what the outcome would be if we applied the pseudo-democratic market process of bidding, buying and selling for short-term profit, if even possible on such a scale, to the creation of high integrity goods systems, such as an airplane, computer, car, home or the like. While today the resources, labor and sub-component systems of these items are certainly in play in the open market, the design itself is not.
The design is relegated, necessarily, to the discipline of science overall. It could be said that a line is intuitively drawn in this way between what is susceptible to monetary opinion and what is tangibly needed to keep some basic level of technical, system integrity. (Please note that this notion of design is not to be confused with subjective “style” interests. Design, as used here, is not an aesthetic consideration but a technical one.)
Imagine, hypothetically, if people “bid and offered” for the physical design construction of a house in each tiny physical detail, ignoring scientific principles. In other words, instead of referencing the basic laws of physics and the natural science that defines the core structural integrity of any building, we let the market decide, with everyone “buying” and “selling” such premises for their personal gain, regardless of their technical understanding. Of course, such an idea is truly absurd in such abstraction and most reading this probably can't even imagine such an irrational interplay.
However, this is exactly what is occurring as a result of our economic system in many other less obvious ways. For example, on the “macroeconomic” scale, the global commercial network created by what is termed globalization[7] - with its basis in cost efficiency which, among other things, utilizes cheaper labor in often distant regions,[8] while wasting large amounts of energy sending resources all over the world and back - reveals this loss of efficiency well.[9]
From the perspective of preconceived design, given the more logical possibility of localization of labor, production and distribution in most all cases, globalization, in its current form, is highly inefficient compared to other possibilities. This is not to deny that globalization and this integration of international economies has generally been a productive occurrence within the evolution of economics. In that context, it has served global industrial development fairly well. However, if we step out of the box of market logic and examine how we could directly design a more technically efficient and localized set of systems, within the global setting, we find that the current method is not only inferior, it is rather offensive.On the “microeconomic” scale, this can be exemplified with respect to the inefficiency inherent to the quality of basic good components, also due to the practice of cost efficiency and the inherent interest to produce the so-called “best” at the “lowest cost” which, quite simply, does not produce the best at all.
For example, a proposed schematic design of, say, a laptop computer might be reasonably efficient, technically. However, if the actual materials used to generate that final good are relatively poor in quality, no matter how intelligent the overall, basic design, it will incur relative weakness and will likely break down more rapidly than if the same design was also optimized to use the most “appropriate” materials from a technical point of view, rather than those materials decided upon as per the proxy of “market efficiency”.[10]Another example is the market phenomenon of proprietary technology. While we see, ostensibly, an enormous amount of variety in the world today with respect to good production, a closer look shows a vast and wasteful multiplicity,[11] along with problematic structural incompatibility between producers' components of the same good genre.
In other words, competing corporations today tend to create custom systems (such as a computer system and its required components) that are incompatible with the developments of other producers in that same good genre. “Universal compatibility”,[12] or lack thereof, is yet another example that the byproduct of this market “proxy” game and the larger order system inefficiency and waste is enormous. This pattern is also common to generational development of existing commercial product systems (aka “models”), such as when improvements are made to a given machine, unnecessarily making obsolete older components of that machine, in the interest of assuring further purchases from the consumer.[13]
It is critical to note that there is no such thing as a single product in the closed system of Earth with respect to planetary resources and their use, nor are any product designs or production methods existing in a vacuum. Each good and its process of production is merely an extension of the whole of industry. Hence, the materials utilized, along with designs, find their true context only with respect to the whole of industry and resource management on all levels. This understanding forces the constant need to view industry (and hence economics itself) as a single system process to ensure maximum technical efficiency.
So with this in mind, coupled again with the first point regarding the question of why certain realities are not put into practice even though they are clearly doable at a given point in time, this essay will examine socially relevant technological trends and design capacities which, if applied properly, could radically transform the world into a “post-scarcity”, highly abundant condition that would alleviate the vast majority of the world's problems we see as commonplace today.
Moreover, it is a conclusion of TZM that the current model not only disallows (or too slowly incorporates) new advents in efficiency due to the very nature of business and its tendency to preserve inefficiency for the sake of an establishment's profit,[14] the very detached and segregated nature of market activity inherently ignores larger order considerations to source and solve problems or accelerate improvement.[15]
Design Efficiency
If we break down the everyday complexity of our lives today, dissecting what interplay is most critical to human survival, sustainability and prosperity, we might find three basic things: science, natural law & resources. 'Science' is the mechanism for discovery and validation; 'natural law' is the pre-existing rule set which we are continually learning about via science and necessarily adapting; while 'resources' exist in the context of both raw Earthly materials and the power of the human mind to comprehend. With respect to the development of design, these three attributes are naturally indispensable to each other.Furthermore, the term industrial design,[16] for the purposes of this essay, will be used to denote the process of economic oriented industry in all its facets, from singular good creation to the total order of the global economy in form. The history of industrial design is, in many respects, the true history of economic development. As our ever-emerging scientific understandings generate logical inference with respect to how best utilize our resources and time, the global landscape, both physical and cultural, has undergone perpetual change.
In this context, the core interest of industrial design is essentially efficiency and it could be argued that there are three central efficiency contexts related: (a) labor efficiency (b) material efficiency and (c) system efficiency.
(a) Labor efficiency has a unique history. Since the early 20th century there has been a relatively rapid transition from the dominant use of human and animal muscle as the source of labor power, to the use of powered machines. This phenomenon, which is termed mechanization, was able to elevate the workforce from much strenuous toil, to operate in more of a position of tool utilization.
However, by the end of the 20th century, this pattern continued to advance, where such machines were not only capable of moving heavy loads and performing complex physical acts, they were also merging with computerization and degrees of artificial intelligence (AI) and hence were able to make decisions as well. In short, the accelerating trend today has proven that these modern machines are now greatly surpassing, in productivity, the vast majority of the actions historically held by human beings and there appears to be no slowing down of this trend.[17] Overall, TZM views this trend as suggesting a powerful means by which the human species can further maximize its productive ability to meet the needs of all human beings, while generating a level of human freedom never before seen, if adapted properly.[18]
(b) Material efficiency is how well we utilize the raw materials of the Earth. Materials science[19] also has a unique history unto itself, with each period of time discovering new patterns and possibilities. Metallurgy, a domain of materials engineering that studies the physical and chemical behavior of metallic elements and their mixtures,[20] was a very important development historically, enabling a vast spectrum of possibility through the creation of compounds and alloys. For example, the term “Bronze Age”,[21] which was the period in Europe of around 3200-600 BC, is characterized by the common use of copper and its alloy bronze for many purposes.
However, perhaps the most important discovery in materials science understanding (and perhaps one of the most important discoveries in human history) was the set of chemical elements that comprise all matter, as we know it. Recognized by most as organized via the “periodic table”, 118 elements have been identified as of 2013, with about 92 known to occur naturally on Earth.[22] In short, these chemical elements are the building blocks of everything we experience as tangible in the world around us and each respective atom has certain properties and hence idiosyncratic applications.
This knowledge, which is extremely new relative to the totality of human understanding,[23] has not only allowed for a deeper understanding of how chemistry can work to create an incredibly vast range of materials for increasingly efficient industrial use, it has also facilitated a powerful understanding of the very nature of matter itself and prospects for manipulation at the atomic scale.
Nanotechnology,[24] which is very much in its infancy, appears quite concrete in its theoretical basis of assembling and disassembling different materials, and even systems of materials (i.e. goods themselves), from the atomic level up and down.[25]
Of course, as profound as that is, the current, relatively crude state of nanotechnology applies mostly in the context of what are called “smart materials”[26] or “meta-materials”.[27] As will be touched upon later in this essay, the current state and trends of materials science hold profound possibilities for the present and future.
(c) System efficiency is likely the most crucial and important of all concepts for, as abstract as it may seem, everything we know of is a system itself or an interaction of two or more systems. Perhaps the best way to express system efficiency is to consider any commonplace act and think about how that act could either reduce waste or increase productivity on any and all levels, not just within the context of the perceived singular act itself. System perspectives are rather obscure to most since we tend to view most functions and processes within the bounds of their intended purpose only, in a categorical manner.
For example, when we consider a modern fitness center (aka “gym”), with people exercising on various machines in one location, we tend to think only of the purpose of that institution and hence how to better facilitate the health interests of those people using those machines, etc. We rarely think more broadly and propose: “What if all those people pedaling and pushing and pulling had that exerted energy run into a conversion system where the building itself could be powered, in whole or in part, by that energy in the form of electricity?”[28]
This manner of thought stands at the heart of a systems theory type worldview. Perhaps a useful way to think of this network perspective is through the synergy of nature itself. In the Earth's biosphere, minus current human interference, there is virtually no such thing as waste. Virtually everything we find in nature is deeply integrated and in balance due to the refining nature of evolution itself.
This is a powerful observation and the term biomimicry[29] is worth mentioning in this context as, in many ways, our development as a species has been to learn from the natural processes in existence already, even though we appear to have decoupled greatly in many ways. Hence, working to facilitate the most optimized integration we can, ideally reusing everything on all levels, just as nature does, should be a societal goal to ensure sustainability and efficiency.
Established and Potential Trends
There are two broad, basic trends/realities to consider in the world today. For the purposes of this essay, we will refer to them as “established” and “potential”. Established trends are the socioeconomic trends in play at the time of this writing and these, in the context of public health and ecological balance, are shown to be almost entirely negative.[30] The potential trends, on the other hand, reveal life-improving and balance-creating possibilities that could occur, if larger order social changes were made. As noted before, these two trends arguably appear to operate in system contradiction of each other.
In the essay titled Social Destabilization & Transition, an in-depth look at the current state of societal affairs will be addressed in detail. However, let it be stated that the efficiencies defined, defended and suggested here are not done so simply to show how much better the world “could be”, as though what we are doing today is still okay. On the contrary, these basic observations actually demand alignment if we intend to maintain stability in our world given its current, degrading patterns.
With a population expected to reach over 9 billion by 2050,[31] with reported trends of looming food,[32] water[33] and energy shortages,[34] these suggestions not only seek to improve but to actually change course. Overall, it is the view of TZM that if these current, so-called established trends persist with the shortsighted market-based practice and all the characteristics that go along with it, human culture will not only not achieve the positive application of the potential trends expressed, increased destabilization will continue to occur.
Post-Scarcity Worldview
In this section, basic statistics and trends will be presented to show how we can, as a global society, achieve a “post-scarcity”[35] social system. While scarcity in absolute terms will always be with humanity to one degree or another in this closed system of Earthly resources, scarcity on the level of human needs and basic material success is no longer a viable defense of the market system's allocation methods.[36] As a brief aside, a common defense of the price system and the market is that if any scarcity exists, it makes void any other approach. The argument goes that since not everyone can have xyz, xyz is scare and hence people need money (or have a lack thereof) to filter out who gains xyz and who doesn't.
The problem with this assumption is that it ignores how certain resources and hence goods have more relevance than others when it comes to public health. Comparing the scarcity of a very expensive, luxury car which draws status satisfaction from its owner more than its basic purpose as a mode of transport - with the scarcity of food, which is a core life requirement for health, is not legitimate in real life terms. The former interest, while perhaps important to the ego satisfaction of the owner who likely already has his or her basic needs met to afford such a product, is not equivalent to the latter interest of those who have little or nothing to eat and hence cannot survive. One cannot arbitrarily conflate such “needs” and “wants”, as though they are simply the same, in theory. Sadly, this is how the market system behaves.
Likewise, with great wealth and material imbalance,[37] comes inevitable social destabilization. Virtually every large-scale public dissent and revolution we have seen in the past couple hundred years have had some economic basis, usually revolving around societal imbalance, exploitation and class separation.[38] The same goes for the roots of crime, terrorism, addictions and other social problems. Virtually all of these propensities are born out of deprivation, whether absolute or relative and this deprivation is inherent to the nature of a society based on competition and scarcity.
So, to simply reduce our economic reality down to mere trade, coupled with the claim that any degree of scarcity justifies the use of the market, price and money for allocation, is to ignore the true nature of what ensures social harmony, stability, and public health. Would it seem reasonable to forgo the technical ability to, say, elevate 80% of humanity to the material capacity currently held by only 10% today, simply because “not everyone can own a 500 room mansion”? Again, the absurdity of this objection is quite clear when a system perspective is taken with respect to what underscores true public health and social stability.
That aside, below is a list of current life support realities available to the global population that have gone unharnessed due to inhibiting factors inherent to the market economy. Each point will be addressed in its own sub-section.
- Food Production: Current production methods already produce more than enough food to feed all human beings on earth. Furthermore, current trends toward more optimized technology and agricultural methods also show a capacity to further increase production efficiency and nutrition quality to a state of active abundance, with minimal human labor and increasingly less energy, water and land requirements.
- Clean Water: Desalination and decontamination processes currently exist to such a vast degree of application that no human being, even in the present state of pollution levels, would ever need to be without clean water, regardless of where they are on earth.
- Energy: Between geothermal, wind, solar, and hydro, coupled with system-based processes that can recapture expelled energy and reuse it directly, there is an absolute energy abundance which can provide for many times the current world's population.
- Material Production/Access: The spectrum of material production, from buildings to transport to common goods, has experienced a powerful merging of capital goods, consumer goods and human labor. With proper system incorporation of each genre of production, coupled with optimized regeneration processes and a total transformation from the use of property rights to a system of access rights, it is clear that all known good functions (in the form of product) can be utilized by 100% of humanity, on a per need basis, in access abundance.
Carrying Capacity
However, before these four issues are addressed in detail, an analysis of the Earth's “carrying capacity” is in order. Carrying capacity is defined as “the maximum, equilibrium number of organisms of a particular species that can be supported indefinitely in a given environment.”[39]Speculation on the Earth's carrying capacity with respect to human beings, meaning how many people the Earth and its biosphere can support, has been a controversial subject for many centuries. For example, a 2001 United Nations report said that two-thirds of the estimates they noted at that time fell in the range of 4 billion to 16 billion with a median of about 10 billion.[40]
However, technological change, and its capacity to increase efficiency with respect to how our resources are used,[41] presents an ongoing interference in such attempts to arrive at a tangible, empirical figure. The reality is that the number of people the Earth can support is highly variable and based, in part, on the current state of technology at a given time and the more we progress our scientific and technical understanding, the more people we tend to be able to support, with less energy and resources applied per person.
Of course, this isn't to imply that within the closed system of the Earth we have some infinite capacity to reproduce. Rather, it highlights the relevance of what it means to be strategic, intelligent and efficient with our resource use and, by extension, the industrial/economic process itself.
Today, there is no evidence that we are at or are closely approaching the Earth's carrying capacity, if we take into account the trends that reveal our vast potential to “do more with less”, coupled with a value system that clearly recognizes that we, as a species, occupy a closed Earth system with natural limitations overall and that it is our personal responsibility to ourselves, each other and future generations to keep an interest in balance, efficiency and sustainability.
This educational imperative suggests that a conscious, informed global culture can stabilize its reproduction rate if need be, without external force, if this basic relationship is properly understood. Of course, much could be said about the influence of old, traditional beliefs, such as religious doctrines that appear to suggest that ongoing and constant procreation is a virtue. These views, which originated in the absence of the knowledge we have today regarding our shared existence on a finite planet, will likely be overcome naturally, with education.[42]
Likewise, if current regions of accelerating population growth are analyzed, it is found that those existing in deprivation and poverty are reproducing faster than those who are not in poverty. While there is some controversy as to why this pattern prevails, the correlation appears to still be accurate. This evidence suggests that increasing people's standard of living can curtail their rates of reproduction and this furthers the social imperative to create a more equitable system of resource allocation.
(1) Food Production
According to The United Nations Food and Agriculture Organization, one out of every eight people on Earth, (nearly 1 billion people) suffer from chronic undernourishment. Almost all of these people live in developing countries, representing 15 percent of the population of these counties.[43] Poverty is, needless to say, clearly linked to this phenomenon.
Yet, politics and business aside, world agriculture today actually produces 17 percent more calories per person than it did 30 years ago, despite a 70 percent population increase. There is enough food to provide everyone in the world with at least 2,720 kilocalories (kcal) per day, which is more than enough to maintain good health for most.[44] [45] Therefore, the existence of such a large number of chronically hungry people in the developing world today reveals, at a minimum, that there is something fundamentally wrong with the global industrial and economic process itself and not the Earth's carrying capacity, or humanity's ability to process enough resources.According to the Institution of Mechanical Engineers, “[W]e produce [globally] about four billion metric tonnes of food per annum. Yet due to poor practices in harvesting, storage and transportation, as well as market and consumer wastage, it is estimated that 30–50% (or 1.2–2 billion tonnes) of all food produced never reaches a human stomach. Furthermore, this figure does not reflect the fact that large amounts of land, energy, fertilizers and water have also been lost in the production of foodstuffs which simply end up as waste.”[46]
In the words of food waste researcher Valentin Thurn, “the number of calories that end up in the garbage in North America and Europe would be sufficient to feed the hungry of this world three times over.”[47]Economically, First World waste patterns can create price increases for the global food supply due to increased demand resulting from those very waste patterns. In other words, the First World adds to the world hunger epidemic by its waste patterns on the consumer end because the resulting demand from increased waste increases price values past what is affordable for many.
While there is certainly an educational imperative for the consuming world to consider the relevance of their waste patterns in the current climate, both in terms of real food waste and its effect on global prices due to increased demand because of this waste, it appears that the most effective and practical means to overcome this global deficiency is to “update” the system of food production itself with modern methods. This, coupled with deliberate localization of the process itself in order to reduce the vast spectrum of waste caused by inefficiencies in the current global food supply chain,[48] would not only reduce such problems in general, it would dramatically increase productivity, product quality and output overall.While the active use of arable land and land-based agriculture should remain (ideally, of course, with more sustainable practices than we are using today),[49] a great deal of pressure can be alleviated at this time with advanced soilless methods, which require less water, less fertilizer, fewer (or no) pesticides, less land, and less labor. These facilities can now be built in urban city environments or even off coastlines, at sea.[50]
Perhaps the most promising of all such arrangements is what is known today as vertical farming.[51] Vertical farming has been put to test in a number of regions, with extremely promising results regarding efficiency. Extrapolating these statistics, coupled with parallel trend advancement (increases in efficiency) of the associated mechanisms of this process, reveals that the future of abundant food production will not only (compared to the current land-based tradition) use fewer resources per unit output, cause less waste, have a reduced ecological footprint, increase food quality and the like; it will also use less of the surface of the planet and enable types of food that where once restricted to certain climates or regions to be grown virtually anywhere in enclosed, vertical systems.
While approaches vary, common methods include rotating crop systems in transparent enclosures to use natural light, coupled with hydroponic,[52] aeroponic[53] and/or aquaponic[54] water and nutrient servicing systems. Artificial light systems are also being used, along with other means to distribute natural light, such as the use of parabolic mirror systems that can move light without electricity.[55] Many “waste to energy”[56] systems approaches to these structures are increasingly common, as with advanced power systems based on regenerative processes or localized sources. Between various approaches, the capacity is dramatically increased since food can be grown almost 24 hours a day, seven days a week.
Common objections to this type of farming have mostly been concerns over its energy footprint, criticizing the use of artificial light in some arrangements as too power intensive. However, the use of renewable energy systems, such as photovoltaics, coupled with regional placement most conducive to renewable methods, such as near wave, tidal or geothermal sources, presents plausible solutions for sustainable, non-hydrocarbon based powering.
However, it is best to think about this in a comparative context. In the U.S., up to 20 percent of the country's fossil fuel consumption goes into the food chain, according to the UN's Food and Agricultural Organization (FAO), which points out that fossil fuel use by the food systems in the developed world "often rivals that of automobiles".[57]
In Singapore, a vertical farm system, custom built in a transparent enclosure, uses a closed loop, automated hydraulic system to rotate the crop in circles between sunlight and an organic nutrient treatment, costing only about $3 a month in electricity for each enclosure.[58] This system is also reported as ten times more productive per square foot than conventional farming, with much less water, labor and fertilizer used, as noted above. There is also no real transport cost given all produce is distributed locally, saving more resources and energy.
Overall, there is a spectrum of applications as of now and, in many cases, these preexisting structures, not intended for such work, are being utilized.[59] In Chicago, IL, USA, the worlds largest certified organic vertical farm is in operation. While producing mostly greens for the local Chicago market, this 90,000 square foot facility uses an aquaponic system,[60] with waste from tilapia fish providing nutrients for the plants. The farm reportedly saves 90% of its water, compared to conventional farming techniques, and produces no agricultural runoff. Additionally, all of its waste, such as plant roots, stems and even biodegradable packaging, is recycled in collaboration, making it a zero-waste facility.[61]
Current statistics vary with respect to the efficiency, often due to monetary-based limitations and inherent profitability concerns. As with much in the market system, promising technology finds development only if it proves competitive. Given how new these ideas are, we cannot expect to see many examples nor can we expect to see an optimization of such methods to a high degree for measurement without “market acceptance”.
However, we can extrapolate the realized potential of existing systems, scaling the application out as if it were incorporated in every major city, in its most relatively efficient form. The following list confirms the superiority of this approach to the current, traditional land based model, not only showing a more sustainable practice, but a more productive practice which can, in concert with existing methods, provide the entire world's population with vegetable based nutrition many times over.[62]
Versatile
Unlike traditional farming, vertical farms can be constructed anywhere, even on water, using upward layers to multiply output capacity. (i.e. a ten-storey farm will produce 1/10 of a 100-storey farm.) This space utilization is limited mostly to architectural possibility. Likewise, the plants grown can be “on demand” in many ways, since region based restrictions have been lifted since these farms can grow virtually anything.
Reduced resource use
Vertical Farming uses substantially less water and pesticide, and is more conducive to non-hydrocarbon based nutrient/fertilizer methods. Its energy use can vary based on application, but in its most efficient setting it uses dramatically less energy both to power the farm itself, and with respect to the now removed need for excess hydrocarbon fertilizer and oil-fueled transport, which is a heavy burden in the current, farm-based process.
More sustainable/less ecological damage
The current tradition of farming has been recognized as one of the most ecologically destructive processes of modern society. In the words of environmental writer Renee Cho:
“As of 2008, 37.7 percent of global land and 45 percent of U.S. land was used for agriculture. The encroachment of humans into wild land has resulted in the spread of infectious disease, the loss of biodiversity and the disruption of ecosystems. Over-cultivation and poor soil management has led to the degradation of global agricultural lands. The millions of tons of toxic pesticides used each year contaminate surface waters and groundwater, and endanger wildlife.
Agriculture is responsible for 15 percent of global greenhouse gas emissions, and accounts for one-fifth of U.S. fossil fuel use, mainly to run farm equipment, transport food and produce fertilizer. As excess fertilizer washes into rivers, streams and oceans, it can cause eutrophication: algae blooms proliferate; when they die, they are consumed by microbes, which use up all the oxygen in the water; the result is a dead zone that kills all aquatic life. As of 2008, there were 405 dead zones around the world...more than two-thirds of the world’s fresh water is used for agriculture.”[63]
Post-scarcity capacity
Students at Columbia University working on vertical farm systems determined that in order to feed 50,000 people, a 30-storey building the size of a “New York City” block would be needed.[64] A New York City block, loosely speaking, is about 6.4 acres.[65] If we extrapolate this into the context of the city of Los Angeles, CA, USA, with a population of about 3.9 million,[66] with a total acreage of about 318,912,[67] it would take roughly 78 thirty-storey, 6.4 covering land acre structures to feed the local residents. This amounts to about 0.1% of the total land area of Los Angeles to feed the population.[68]
The Earth, being about 29% land, has roughly 36,794,240,000 acres and a human population of 7.2 billion as of late 2013.[69] If we extrapolate the same basis of a 30-storey vertical farm covering 6.4 acres to feed 50,000 people, we end up needing 144,000 vertical farms, in theory, to feed the world.[70] This amounts to 921,600 acres of land to place these farms.[71] Given roughly 38% of all the Earth's land is currently being used for traditional agriculture (13,981,811,200 acres),[72] we find that we need only 0.006% of the Earth's existing farmland to meet production requirements.[73]
Now, these extrapolations are clearly theoretical and obviously many other factors need to be taken into account with respect to placement of such farm systems and critical specifics. Also, within the 38% land use statistic, much of that land is for livestock cultivation, not just crop production. However, the raw statistics are quite incredible with respect to possible efficiency and capacity. In fact, if we were to theoretically take only the crop production land alone currently being used, which is about 4,408,320,000 acres,[74] replacing the land based cultivation process by placing these 30-storey vertical farm systems only (side by side), the food output would be enough to feed 34,440,000,000,000 people.[75] (34.4 Trillion)
Given that we will only need to feed 9 billion by 2050, we only need to harness about 0.02% of this theoretical capacity, which, it could be argued, likely makes rather moot any seemingly practical objections common to the aforementioned extrapolation. As a final note, proteins, which are readily available in the vegetative realm, are still brought into question in the modern day with respect to interest in meat production. From a sustainability standpoint, ignoring the common moral issues and arguably inhumane practices still common to industrialized livestock cultivation, the production of meat is an environmentally unfriendly act today.
According to the ILRI, livestock systems occupy about 45% of the Earth's surface.[76] According to the FAO, livestock sector produces more greenhouse gas emissions than modern gas consuming transport.[77] Given that 90% of all the large fish once thriving in the ocean are gone due to overfishing as well,[78] new solutions are needed.
One such solution is aquaculture, which is the direct farming of fish, crustaceans and the like. This direct approach, if sustainably driven, can provide farm raised, protein rich fish for human consumption, replacing the demand for land-based meat. Another approach is the production of “in vitro meat”. In vitro meat may be produced as strips of muscle fibre, which grow through the fusion of precursor cells, either embryonic stem cells or specialized satellite cells found in muscle tissue. This type of meat is usually cultured in a bioreactor.
While still experimental, in 2013 the world's first lab-grown burger was cooked and eaten in London.[79] Other benefits include the reduction of livestock sourced disease which is very common, along with being able to avoid certain negative health characteristics of traditional meat, such as the removal of fatty acids in production.
(2) Clean Water
Given that the human body can only survive a few days without fresh water,[80] making this most basic resource abundantly available to all is critical. Likewise, it is the backbone of many industrial production methods, including agriculture itself. Fresh water is naturally occurring water on the Earth's surface in ice sheets, ice caps, glaciers, icebergs, bogs, ponds, lakes, rivers and streams, and underground as groundwater in aquifers and underground streams. Of all the water on Earth, 97% of it is saline and not directly consumable.
According to the World Health Organization: “About 2.6 billion people – half the developing world – lack even a simple ‘improved’ latrine and 1.1 billion people have no access to any type of improved drinking source of water. As a direct consequence:
- 1.6 million people die every year from diarrhoeal diseases (including cholera) attributable to lack of access to safe drinking water and basic sanitation and 90% of these are children under 5, mostly in developing countries;
- 160 million people are infected with schistosomiasis causing tens of thousands of deaths yearly; 500 million people are at risk of trachoma from which 146 million are threatened by blindness and 6 million are visually impaired;
- Intestinal helminths (ascariasis, trichuriasis and hookworm infection) are plaguing the developing world due to inadequate drinking water, sanitation and hygiene with 133 million suffering from high intensity intestinal helminths infections; there are around 1.5 million cases of clinical hepatitis A every year.”[81]
According to the United Nations, by 2025, an estimated 1.8 billion people will live in areas plagued by water scarcity, with two-thirds of the world's population living in water-stressed regions.[82] As with most all of the world's current resource problems, it is an issue of both poor management and a lack of industrial application. From the standpoint of management, the amount of water wasted in the world due to pollution, overuse and inefficient infrastructure is enormous. About 95% of all water that enters most people's homes goes back down the drain in one shot.[83] A systems-based solution to optimize this use is to design kitchens and bathrooms so they recapture water for different purposes. For example, the water running through a sink or shower can be made available for a toilet. Various companies have slowly put such ideas to practice recently but overall most infrastructures do nothing of the sort as far as reuse schemes. The same is true of large commercial buildings, which can create reuse networks throughout the whole structure, coupled with capture of rainwater for other purposes, etc.Water pollution is another problem, which affects both developed and developing nations on many levels. The American Environmental Protection Agency (EPA) estimates that 850 billion gallons of untreated discharges (waste) flow into water bodies annually, contributing to over 7 million illnesses each year.[84] The Third World Centre for Water Management estimates that only about 10 to 12 percent of wastewater in Latin America is treated properly. Mexico City, for example, “exports” its untreated wastewater to local farmers. While the farmers value this because the water increases crop yields, the wastewater is heavily contaminated with pathogens and toxic chemicals, representing a serious health risk for both farmers and consumers of the agricultural products grown in this area. In India, major cities discharge untreated wastewater into the bodies of water that serve as their drinking water. Delhi, for example, discharges wastewater directly into the Yamuna River—the source of drinking water for some 57 million people.[85]Solutions to this problem, in part, must address the issue of the vast inefficiency, likely driven by the monetary limitations of most governments, to institute proper waste systems, coupled with an industrial design imperative to include reuse system techniques to better preserve and utilize our existing resources.That aside, the most notable, broad solution to compensate for these emerging problems, to facilitate not only an alleviation of the current water problems affecting over 2 billion people but also to transcend into a condition of relative abundance of fresh water for all humans, is to utilize modern (a) purification and (b) desalination systems both on the macro-industrial and micro-industrial scale.
(a) Purification
Advancements in water purification have been accelerating rapidly with many technological variations of approach. Perhaps one of the most efficient today is what is called “ultraviolet (UV) disinfection”. This process is highly scalable, low energy and works quickly. According to engineer Ashok Gadgil, inventor of portable UV systems, “In terms of energy use, 60 watts of electrical power – which is comparable to the power used in one ordinary table lamp – is enough to disinfect water at the rate of one ton per hour, or fifteen liters per minute...This much water is enough to meet the drinking water needs of a community of 2,000 people.”[86] This device Gadgil developed for rural, poor areas can run off of solar panels and weighs only 15 pounds and has no toxic discharge.Of course, there is no silver bullet. While UV disinfection works very well for bacteria and viruses, it is less effective with other types of pollution such as suspended solids, turbidity, color, or soluble organic matter.[87] In large-scale applications, UV is often combined with more standard treatments, such as chlorine, as is the case with the world's largest UV drinking water disinfection plant in New York, which can treat 2.2 billion US gallons (8,300,000 cubic meters) per day.[88] That is 3,029,500,000 cubic meters a year.The average person in the United States uses 2842 cubic meters a year.[89] This includes fresh water used for industrial purposes, not just for direct (drinking) consumption. The global average is 1385 cubic meters per year.[90] China, India and the United States are currently the largest freshwater users in the world and the majority of that water is used in production, mainly agriculture.[91] In fact, about 70% of all freshwater is used for agriculture globally.[92] For the sake of pure statistical argument, ignoring the highly needed revisions towards strategic water use, reuse systems and conservation possibilities through more advanced and efficient industrial applications, let us assess the simple question of what it would require to disinfect (assuming it was needed) all the fresh water currently being used in the world on average by the population, in all contexts. Given the global average of 1385 cubic meters and a population of 7.2 billion, we arrive at a total annual use of 9.972 trillion cubic meters.Using the New York UV plant's output capacity of roughly 3 billion cubic meters a year as a base per installation of such a plant, we find that 3,327 plants would be needed globally.[93] The New York plant is about 3.7 acres (160k sq. ft.).[94] This means about 12,309 acres of land is needed, in theory, to facilitate a purification process of all the fresh water currently used globally by the population. Of course, needless to say, there many other “footprint” factors that come into play, such as power needs, coupled with the critical importance of location.However, let's put this into a larger, more thoughtful comparison. The United States military alone, with its roughly 845,441 military buildings and bases, occupies about 30 million acres of land globally.[95] Only 0.04% of that land would be needed to disinfect the total fresh water use of the entire world, if it were even needed at scale, which it is not.
(b) Desalination
The realistic possibility of mass, global purification of polluted fresh water aside, likely the most powerful means to assure usable, potable water is to convert directly from a saline source, namely the ocean. With a planet comprised of mostly salt water, this technique, if done properly, assures global abundance alone.The most common method of desalination used today is reverse osmosis, a process that removes water molecules from salt water, leaving salt ions in a leftover brine waste by-product. According to the International Desalination Association: “Currently, reverse osmosis (RO)...accounts for nearly 60 percent of installed capacity, followed by the thermal processes multi-stage flash (MSF) at 26 percent and multi-effect distillation (MED) at 8.2 percent.”[96] As of 2011, there were roughly 16,000 desalination plants worldwide, and the total global capacity of all plants online (e.g., in operation) was 66.5 million cubic meters per day, or approximately 17.6 billion US gallons per day.[97] As with everything technological, many advancing methods currently considered “experimental” suggest a powerful increase in efficiency as the trends unfold. One such method called capacitive desalination (CD), also known as capacitive deionization (CDI), has been shown to operate with greater energy efficiency, lower pressures, no membrane components and it does not produce a waste discharge like conventional practices. It can also be easily scaled-up simply by increasing the number of flow electrodes in the system.[98]Overall, if we examine the existing methods in general, coupled with emerging methods, we see a general trend of increasing efficiency in both power conservation and performance.[99] That briefly noted, the focus of this extrapolation towards a “post-scarcity” utilization of desalination will consider only current, proven, in-use methods, namely the reverse osmosis system.
The Wonthaggi Desalination Plant is an advanced reversed osmosis seawater desalination plant on the Bass Coast near Wonthaggi, in southern Victoria, Australia. It was completed in December 2012. It can produce, conservatively, about 410,000 cubic meters of desalinated water a day (150 million cubic meters a year),[100] while occupying about 20 hectares (about 50 acres of land).[101] Since, as noted before, the total, annual water use of the world today is about 9 trillion, 972 billion cubic meters, this means that it would take 60,000 plants[102] to process all potable water usage. Once again, this extreme extrapolation is to make a relative point, since we do not need to desalinate that much water in reality.However, assuming that we did need to desalinate seawater constantly to match current global use, 3 million acres of land would be needed total. Earth has about 217,490[103] miles of coastline which means, loosely using the Wonthaggi model of roughly 20 hectares (roughly 50 acres) with 100 meters per hectare (or 328 feet), assuming the construction was four hectares deep and five hectares long, parallel to the coastline, the plant would take up 1640 feet along the coast. This means, assuming 60,000 plants of the same dimension, it would take up 98,400,000 feet or 18,636 miles of coastline (8.5% of the world's coastline).Of course, that is a great deal of coastline and naturally many other factors come into play when choosing an appropriate location for such a plant. Again, it is not the purpose of this extrapolation to suggest these statistics are of any other use than to gauge a broad sense of what such capacity means, in light of the water scarcity/stress issues occurring today. Yet, the fact is, it is clearly within the range of such application to meet the needs of people suffering from water scarcity via desalination alone, coupled with an infrastructure and distribution system to move water inland.As a final example, let's reduce this abstract extrapolation more so and apply it to a real life circumstance. On the continent of Africa, for example, which has about 1 billion people as of 2013,[104] roughly 345 million people lack sufficient access to potable water.[105] If we apply the noted global average consumption rate of 1385 cubic meters a year, seeking to provide each of those 345 million people that amount, we would need 477,825,000,000 cubic meters produced annually.
Using the Wonthaggi annual capacity of 150 million cubic meters produced as the base figure, Africa would need 3185 50-acre plants along its coast line to meet such demand, taking up about (25,158 miles of coastline in Africa) 5,223,400 feet or 989 miles. This takes up only 3.9% of Africa's coastline.[106]
However, if we divided this number in half, and used UV purification systems for one section and desalinization for the other, the desalinization process would need about 1.9%, or 494 miles of coastline for desalination facilities and only about 296 acres of land for purification facilities, which is a minuscule faction of Africa total landmass (of about 7 billion acres). This is highly doable and obviously, in this case and in all cases, we would strategically maximize purification processes since it is more efficient, while using desalination for the remaining demand.Such crude statistics reveal that between UV and traditional decontamination, coupled with traditional desalination processes, as they currently exist, even ignoring the rapid advancements occurring in both fields[107] which will likely have an exponentially advanced level of efficiency in the coming decades, the idea of enduring water scarcity on planet Earth is absurd. Both of these isolated extrapolations have assumed only one or the other was applied, in only large scale form, assuming there are no other existing sources of potable water. In reality, given the existing level of freshwater still available, coupled with a simple, intelligent re-ordering of use-reuse water network schemes to further preserve the existing capacity, coupled with both large and small scale desalination and decontamination processes as regions require (many of which can be powered by rapidly advancing renewable energy processes as well), we have the technical capacity to bring potable water availability to absolute global abundance.
(3) Energy
Renewable energy sources are sources that are continually replenished. Such sources include energy from water, wind, solar and geothermal. In contrast, fuels such as coal, oil, and natural gas are non-renewable as they are based on Earth stores which show no near-term regeneration.
As of the early 21st century, the recognition of clean, renewable energy possibilities has been substantial.[108] The spectrum of application, scalability and degree of efficiency, coupled with advancing methods for energy storage and transfer, have arguably made our current, mostly hydrocarbon-based energy methods appear outdated, especially given the ongoing negative consequences of their use. Nuclear energy, while effective and considered a “renewable” form by some, works at very high risk given the unstable materials involved and the large scale accidents on record have brought the safety of this form of production into question as well.[109]
In the world today, the five most commonly used renewable sources are hydropower (via dams), solar, wind, geothermal and biofuels. Renewable energy sources currently represent about 15% of global energy use, with hydro-power accounting for 97% of this figure.[110]
Given that over 1.2 billion people are without access to electricity worldwide,[111] coupled with the ongoing pollution and periodic crises associated with traditional, non-renewable methods, the purpose of this subsection is to show how the dangerous realities associated with fossil fuels and nuclear energy are no longer needed. We can now power the world many times over with clean, renewable, relatively low impact methods, largely localized as per the needs of a single structure, city or industrial application.
However, it is important to point out upfront that there is no single solution at this time. Given that different areas of the Earth have different propensities for renewable energy harnessing and use, application must be seen as a system or network development of a combination of mediums. That noted, narrowing down the most relevant of these abundance producing possibilities, it is perhaps best to think of renewable energy extraction/harnessing and use in two categories: (a) Large scale/Base-load & (b) Small Scale & Total Mixed-Use systems.
(a) Large Scale/Base-load
Large-scale generation, such as for “base-load” needs required to power a city or high energy industrial center, includes four main mediums: (a1) geothermal plants, (a2) wind farms, (a3) solar fields, and (a4) water (ocean/hydropower).
(a1) Geothermal:
Geothermal power[112] is energy harnessed essentially from the natural heat of the Earth's molten core, with plants usually placed around areas where the distance to large heat centers is fairly shallow.[113] [114] A 2006 MIT report on geothermal energy, promoting an advanced extraction system called EGS, found that 13,000 zettajoules of power are currently available in the Earth, with the possibility of 2000 zettajoules being harvestable with improved technology.[115]
The total energy consumption of all the countries on the planet is about half a zettajoule (0.55) a year[116] and this means thousands of years of planetary power could be harnessed in this medium alone. The MIT report also estimated that there was enough energy in hard rocks 10km below the US alone to supply all the world's current needs for 30,000 years.
Even with an expected 56% increase of consumption by 2040, geothermal's capacity is enormous if property tapped.[117] [118] Likewise, the extraction of heat taking place from within the Earth appears quite minor in comparison to its store, making the source virtually limitless in proportion to actual human consumption.[119] Also, since the energy is produced constantly, there are no intermittency problems and this type of energy can be produced constantly without the need for storage.
The environmental impact of geothermal is relatively very low. Iceland has been using it almost exclusively for some time and their plants produce extremely low emissions (no carbon) when compared to hydrocarbon-based methods.[120] Apart from some sulphur produced, small earthquakes can occur as a result of drilling techniques. This problem has been acknowledged as human induced[121] and improvement in the engineering process is the solution, coupled with clear understanding of the nature of the location for drilling.As far as location, it is theoretically possible to place geothermal energy extraction plants anywhere, if the capacity to drill deep enough was there, coupled with other advancements in technology.[122] However, today most plants need to exist near where tectonic plates[123] meet on Earth. A geothermal map of the surface of the Earth taken by satellite can show such ideal spots very clearly based on heat emitted.[124] These maps show possibilities near most coastlines around the world[125] and while most studies are ambiguous with respect to exactly how many locations could be made available, the potential recognized, in general, is enormous.
The U.S. Department of Energy has noted that geothermal energy also uses much less land than other energy sources, including fossil fuel and currently dominant renewables. Over 30 years, the period of time commonly used to compare the life cycle impacts from different power sources, a geothermal facility uses 404 meters squared of land per gigawatt hour, while a coal facility uses 3,632 meters squared per gigawatt hour.[126] If we were to do a basic comparison of geothermal to coal given this ratio of meters squared to gigawatt hour, we find that we could fit about nine geothermal plants in the space of one coal plant.[127] [128]
Likewise, it is important to note that new, more efficient methods to tap geothermal appear to be just starting with respect to possible output potential. In 2013 it was announced that a 1000 MW power station was to begin construction in Ethiopia.[129] A megawatt is a unit of power, and power capacity is expressed differently from energy capacity, which is expressed, in this context of megawatts, as megawatt hours (MWh). Put another way, energy is the amount of work done, whereas power is the rate of doing work. So, for example, a generator with one MW capacity that operates at that capacity consistently for one hour will produce 1 MW-hour (MWh) of electricity.
This means if a 1000 MW geothermal power-station operated at full capacity 24 hours a day, seven days a year (365 days), it would produce 8,760,000 MWh/year.[130] The world's current annual usage in MWh is about 153 Billion,[131] which means it would take, in abstraction, 17,465 geothermal plants to match global use.[132]
According to the World Coal Association, there are over 2,300 coal power plants in operation worldwide.[133] Using the aforementioned plant size/capacity comparison of about nine geothermal plants fitting into one coal plant, the space of 1,940[134] (or 84% of the total in existence) coal plants would be needed, in theory, to contain the 17,465 geothermal plants. Also, given coal today accounts for only 41% of the world's current energy production,[135] this theoretical extrapolation also shows how in 84% of the current space usage by coal plants alone (which only produce 41%), geothermal could supply 100% power capacity as per global use instead.
All this without the pollution from coal, which has been considered one of the most polluting practices in the world along with being likely the largest contributor to the human-made increase of CO2 in the atmosphere.
(a2) Wind FarmsU.S. Department of Energy studies have concluded that wind harvesting in the Great Plains states of Texas, Kansas, and North Dakota could provide enough electricity to power the entire USA.[136] More impressively, a 2005 Stanford University study published in the Journal of Geophysical Research found that if only 20% of the wind potential on the planet was harnessed, it would cover the entire world’s energy needs.[137]
In corroboration, two more recent studies by unrelated organizations published in 2012 calculated that with existing wind turbine technology the earth could produce hundreds of trillions of watts of power. This, in effect, is many more times what the world currently consumes.[138] Wind power is perhaps one of the most simple and low impact forms of renewable energy and its scalability is limited only to location.Using the 9,000-acre Alta Wind Energy Center California as a basis, which has an active capacity of 1,320 MW of power, a theoretical annual output of 11,563,200 MWh is possible.[139] This means 13,231 9000-acre wind farms would be needed to meet the current output figure of 153 billion MWh. This means 119,079,000 acres of (wind sufficient) land would be required.[140] This amounts to 0.3% of the Earth's surface that would be needed to power the world, in abstraction.[141] Once again, this is not to suggest such a thing is ideal given what land is feasible for wind farms, along with other important factors. This is simply to give a general perspective of possibility.
However, one unique reality of wind power generation is the potential of offshore harnessing. Compared to land-based wind power, offshore wind power has, on average, a much larger yield, as wind speeds tend to be higher. This reality also alleviates land-based pressures given land scarcity and regional restrictions.
According to the Assessment of Offshore Wind Energy Resources for the United States, 4,150 gigawatts (4,150,000 MW) of potential wind turbine capacity from offshore wind resources are available in the United States.[142] Assuming this power capacity was consistent for a year, we end up with an energy conversion of 36,354,000,000 MWh/yr. Given the United States, in 2010, used 25,776 TWh of energy (25.78 billion MWh),[143] we find that offshore wind harvesting alone exceeds national use by about 10.6 billion MWhs, or 41%.Intuitively, extrapolating this national level of capacity to the rest of the world's coastlines, also taking into account the aforementioned land-based only statistic research that found we can power the world many times over onshore as well,[144] the possibilities of wind-based energy abundance is exceptionally impressive.
(a3) Solar Fields
The upper atmosphere of Earth receives about 1.5 × 1021 watt-hours of solar radiation annually. This vast amount of energy is more than 23,000 times that used by the human population of the planet.[145] If humanity could capture one tenth of one percent of the solar energy striking the Earth we would have access to six times as much energy as we consume in all forms today, with almost no greenhouse gas emissions. The ability to harness this power depends on the technology and how high the percentage of radiation absorption is.
Conventional photovoltaics, currently the most common form used mostly for smaller applications, use silicon as the semiconductor and exist in something of a flat cell or sheet. Concentrated photovoltaics (CPV) are generally more efficient than non-concentrated on average; however they tend to require more direct exposure to focus the light properly.
Concentrated solar power (CPS) is a large-scale approach that uses mirrors or lenses to concentrate a large area of sunlight, or solar thermal energy, onto a small area. Electrical power is produced when the concentrated light is converted to heat, which drives a heat engine (such as a steam turbine) connected to an electrical power generator or the like. Unlike photovoltaics, which convert directly to electricity, this technology converts to heat. Recently, large-scale storage methods have also been used to prolong access at night.
A variation of CPS is STE, or Solar Thermal Energy. The Ivanpah Solar Electric Generating System in California, USA is a 3500 acre field[146] with a stated annual generation of 1,079,232 MWh.[147] While Ivanpah does not use any form of storage, it serves about 140,000 homes in the region. If we were to extrapolate using Ivanpah as a basis, it would take 141,767 fields or 496,184,500 acres to theoretically meet current global energy use based on output. This is 1.43% of total land on Earth.[148]
Once again, this is not to suggest such a thing is practical nor is it to ignore the radiation yield differences found on different areas of the Earth. However, deserts, which tend to be highly conducive for solar fields while often less conducive to life support for people, are roughly 1/3 of all the land mass in the world or about 12 billion acres. Compared to the roughly 500 million acres theoretically needed to “power the world” as per our extrapolation, only 4.1% of the world's desert land would be needed.[149]
Likewise, other projects similar to the Ivanpah field have been incorporating storage systems. The Solana 280MW solar power plant in Arizona combines parabolic trough mirror technology with molten salt thermal storage and is able to continue outputting up to six hours after the sky goes dark.[150]
In general, the rate of advancement of photovoltaic, solar thermal, storage methods and other existing and emerging technologies continue to rapidly advance, revealing that many installations seen as highly efficient today will be grossly inefficient in a decade or two. As will be addressed more so with respect to smaller scale renewable energy solutions, the use of solar power localized in the very construction of buildings and domiciles is likely to be where true future efficiency will take place. The issue is making the technology compact and efficient enough for localized, per case use.However, solar field power stations, just like geothermal and wind, have an enormous global potential in and of themselves and there is little doubt that given proper resources and attention, these fields alone could theoretically establish an infrastructure and efficiency level to power the world alone.
(a4) Water/Hydro EnergyWater-based renewable energy extraction could generally be said to have two broad sources: the ocean itself and river-type water flows which use the gravitational force of falling or flowing water, usually in an inland watercourse. The latter is generally referred to in practice as hydroelectric and, as noted before, it is currently a fairly large part of the existing renewable energy infrastructure.[151]
On the other hand, the vast potential of the ocean has yet to be harnessed within a fraction of its capacity. It is not far-fetched to suggest that the intelligent harvesting of both the various mechanical movements of ocean water coupled with exploiting the differences in heat, known as ocean thermal energy conversion (OTEC), that ocean water power couldn't also power the world alone.[152] [153] [154] Given the existing, fairly large-scale use of hydroelectric power (dams) already, this section will instead focus on the ocean potentials.
The most pronounced sea-based potentials at this time appear to be wave, tidal, ocean current, ocean thermal and osmotic. Waves are primarily caused by winds; tides are primarily caused by the gravitational pull of the moon; ocean currents are primarily caused by the rotation of the Earth; ocean thermal results from solar heat absorbed by the surface of the ocean; and osmotic power is when fresh water and salt water meet, exploiting the difference in salt concentration.
Wave:
It has been found that wave power's usable global potential is about 3 TW[155] or about 26,280 TWh/yr assuming constant harnessing. This is almost 20% of current global use. This amount of power has been ascertained essentially by analyzing deep-water regions off continent coastlines. The theoretical power estimate has been estimated at 3.7 TW, with the final net estimate reduced by about 20% to compensate for various inefficiencies related to a given region, such as ice coverage. Energy output is basically determined by wave height, wave speed, wavelength, and water density.
Wave farms, or the construction of wave harnessing plants off a coastline, have seen limited large-scale application at this time, with only about six countries sparsely applying the technology.[156] Locations with the most potential include the western seaboard of Europe, the northern coast of the UK, and the Pacific coastlines of North and South America, Southern Africa, Australia, and New Zealand.
Tidal:
Tidal has two sub-forms: range and stream. Tidal range is essentially the “rise and fall” of areas of the ocean. Tidal streams are currents created by periodic horizontal movement of the tides, often magnified by the shape of the seabed.
Different locations of Earth have large differences in range.[157] In the United Kingdom, an area with high levels of tidal activity, dozens of sites are currently noted as available, forecasting that 34% of all the UK’s energy could come from tidal power alone.[158] Globally, older studies have put tidal capacity at 1800TWh/yr.[159] More recent studies have put the theoretical capacity (both range and stream) at 3TW, assuming only a portion would be extractable.[160]
Tidal, while very predictable, is also subject to daily periods of intermittency based around tidal shifts. Assuming only 1.5 TW could be harnessed in a year based on advanced technology, this means about 7% of the world's power could come from tidal.
Ocean Current:
Similar to tidal streams, ocean currents have shown great potential. These currents flow consistently in the open ocean and various emerging technologies have been developing to harness this largely untapped medium. As with all renewables, the capacity to harness such potential is directly related to the efficiency of the technology employed. The EOEA estimates the current potential at 400 THW/yr.[161] However, there is good reason to assume this figure is outdated. Prior applications of turbine/mill technologies to capture such water flows have needed an average current of five or six knots to operate efficiently, while most of the Earth's currents are slower than three knots.[162] However, recent developments have revealed the possibility to harness energy from water flows of less than two knots.[163] Given this potential, it has been suggested that ocean current alone could power the entire world.[164]
The Gulf Stream[165] potential has been estimated at 13GW of actual output, assuming a 30% conversion efficiency using more traditional turbine technology.[166] This means 13,000 MW or, assuming constant harnessing of the stream all year, about 113,880,000 Mwh/yr.[167] The United States, in 2011, is estimated to have used 4.1 billion MWh in electricity.[168] This means 30%[169] of the US's electrical consumption could be generated by the Gulf Stream alone. Once again, this is assuming the use of only established technology.
Osmotic:
Osmotic power or salinity gradient power is the energy available from the difference in the salt concentration between seawater and river water. The Norwegian Center for Renewable Energy (SFFE) estimates the global potential to be about 1,370 TWh/yr.[170] with others putting it at around 1,700 TWh/yr[171] or the equivalent of half of Europe's entire energy demand.[172]
While still largely in its infancy, osmotic power harnessing through advancing technology is promising. Power plants can, in principle, be built anywhere freshwater meets seawater. They can generate power 24/7, regardless of weather conditions.Ocean Thermal:The final ocean-based means for energy harnessing worth noting is ocean thermal energy conversion (OTEC). Exploiting the differences in heat existing around the surface of the ocean and below, warmer surface water is used to heat a fluid, such as liquid ammonia, converting it into vapor, which expands to drive a turbine which, in turn, produces electricity. The fluid is then cooled using cold water from the ocean depths, returning it into a liquid state so the process can start all over again.
Of all the ocean-based energy sources, OTEC appears to have the most potential. It has been estimated that 88,000 TWh/yr could be generated without affecting the ocean's thermal structure.[173] While this figure may not express total, usable capacity, it implies that well over half of all current global energy consumption could be met with OTEC alone. As of 2013, most of the existing OTEC plants are experimental or very small scale. However, a few major industrial capacity projects have been set in motion, including a 10 MW plant off the coast of China[174] and a 100MW near Hawaii.[175] One 100MW offshore plant can theoretically power Hawaii’s entire Big Island alone,[176] meaning 186,000 people as of a 2011 census.
Now, in conclusion to this subsection of ocean energy harnessing, keeping consistent with the prior categorical estimations set forward for solar, wind and geothermal, it is worthwhile to consider the total, combined (largely conservative) potential of each noted medium. While this will, of course, be a crude extrapolation since there are many complex variables, including the fact that some applications are still semi-experimental and difficult to properly assess, this general figure still helps to digest the broadest perspective of the potential of ocean renewables. Here is a list of the noted global potentials:
Wave: 27,280 TWh/yr
Tidal: 13,140 Twh/yr (1.5 TW x 8760hr )
Ocean Current: 400 Twh/yr (old estimate with old tech)Osmotic: ~1,500 TwH/yr (average of noted statistics)Ocean Thermal: 88,000 Twh/yr
Added together we arrive at 130,320 TWh/yr or 0.46 ZJ a year. This is roughly 83% of current global use (0.55 ZJ). It is important to note that such numbers are derived, in part, from traditional technologies, with no adjustment made for more recent improvements. If we bring traditional Hydroelectric (watercourse based) back into the equation, which, according to the IEA has a potential of 16,400 TWh/yr,[177] this brings the figure up to 146,720 TWh/yr or 96% of current global use.
(b) Small Scale & Total Mixed-Use Systems
The prior section described the vast potential of large-scale, base-load renewable energy harnessing. Wind, solar, water/hydro and geothermal have all shown that they are capable, individually, of meeting or vastly exceeding the current 0.55 ZJ annual global energy consumption at this time.
The true question is how are such methods to be intelligently put into practice. Given the regional limitations coupled with other native issues such as intermittency, the real design initiative to create a workable combination of such means is needed. Such a systems approach is the real solution, harmonizing an optimized fraction of each of those renewables to achieve global, total use abundance.
For example, it is not inconceivable to imagine a series of man-made floating islands off select coastlines which are designed to possibly harness, at once, wind, solar, thermal difference, wave, tidal and ocean currents – all at the same time and in the same general area. Such energy islands would then pipe their harvest back to land for human use. Various combinations could also be applied to land-based systems as well, such as constructing wind/solar combinations to compliment the fact that often wind is more present at night, while solar is more present during the day.
Likewise, creative ingenuity with respect to how we can intelligently combine various methods also extends to what we could consider localized energy harnessing. Smaller scale renewable methods that are conducive to single structures or small areas find the same systems logic regarding combination. These localized systems could also, if need be, connect back into the larger, base-load systems as well, revealing a total, mixed medium integrated network.
A common example today is the use of single structure solar panels, such as for home use. While the efficiency of these panels is still improving, coupled with imposed cost limitations as per the investment/profit mechanism of the market, most people utilizing these solar power systems are only able to compliment their home's electricity use rather than gain 100% utilization. (For example, most systems are applied to power the home during the day, while pulling power from the regional base-load grid at night.) This kind of approach that seeks to maximize localized possibilities first, before resorting to larger scale energy use, in a system approach, is the key to practical energy abundance, efficiency and sustainability.
To understand the relevance of this more thoroughly, let's expand the example of household solar array application to it possible theoretical potential. In 2011, the average annual electricity consumption for a U.S. residential utility (household) customer was 11,280 kWh.[178] Given 114,800,000 households in 2010,[179] this means 1295 TWh/yr was used. Total electrical energy consumption in 2012 for the USA was 3,886,400,000 MWh/yr.[180] This equates to 3,886 TWh/yr. This means 33% of all electric consumption occurred in people's homes, with the vast majority of that energy coming from fossil fuel power stations.
If all households in the United States were able to power themselves for electricity using solar panels alone, localized energy utilization that is simply wasted at this time, the base-load stress reduction would be dramatic. Contrary to popular belief, as of 2013 this is a real possibility given the state of solar cell efficiency and storage technology.[181] The problem is that the current energy industry is not prepared for such efficiency and consumer solar systems available suffer from high financial expense as a result of limited mass production, competition and a lack social initiative to forward advancement.
It worth stating here that the financial system and its price oriented mechanisms exist as barriers to ubiquitous and optimized household solar development in the broad view (along every other developing technology after a certain point of proven efficacy). While defenders of capitalism argue that the process of investment-to-market of an in-demand good generally reduces the cost of that good over time, making it more available to those who could not afford it before, it is forgotten that the entire process is a contrivance.
If price and profit were removed from the system, focusing only on the technology and its statistical merit, both at the current time and its longer term efficiency trends (future improvements), proper resource allocation strategies and research could be employed to bring promising technology to the population much more rapidly. In the case of solar arrays for home power generation, given the incredible capacity it has to alleviate base-load energy stress which would, today, further reduce emissions and fossil fuel pollution, it is a very unfortunate circumstance this technology and its application is subject to the whims of the market.[182]
If we survey the commercial expense of an average solar array as of 2013, an average home using 11,280 kWh a month would require about 30 panels, with a solar cell efficiency of about 9-15% and a night time battery system. This would cost well over $20,000.[183] Such an expense is unaffordable for the vast majority of the world, even though the basic materials used in traditional PV systems are simple and abundant, along with ever-increasing manufacturing ease.
Likewise, it is equally as disappointing to notice how modern home construction has made little to no use of other basic, localized renewable methods that can further facilitate the real world capacity to bring all households (not only in the USA but the in the world) to a place of energy independence.
Noting the power of solar, other nearly universal applications also apply. Small wind harvesting systems[184] and geothermal heating and cooling technology,[185] combined with architectural design making better use of natural light and heat/cool preservation efficiency,[186] there is a spectrum of design adjustments which could make apartments and houses not only self-sufficient, but more ecologically sustainable. Coupling this with use-reuse designs for water preservation, along with other approaches to optimize energy/resource efficiency, it is clear that our current methods are enormously wasteful when compared to the possibilities.
Extending outwards to city infrastructure, we see the same failures almost everywhere with respect to such applied systems. For example, an enormous amount of energy is used in the process of transportation. While the electric vehicle has proven viable for full global use, even though lobbying efforts and other market limitations have continued to keep its application well behind the gasoline powered norm, many system-based methods also go unharnessed.Apart from a general necessity to reorganize urban environments to be more conducive to convenient mass transit networks, removing the need for numerous autonomous vehicles, simply re-harnessing the powered movements of all transport mediums could dramatically alleviate energy pressures.
A technology called piezoelectric,[187] which is able to convert pressure and mechanical energy into electricity, is an excellent example of an energy reuse method with great potential. Existing applications have included power generation by people walking on piezo engineered floors[188] and sidewalks,[189] streets which can generate power as automobiles cross over them[190] and train rail systems which can also capture energy from passing train cars through pressure.[191] Aerospace engineer Haim Abramovich has stated that a stretch of road less than a mile long, four lanes wide, and trafficked by about 1,000 vehicles per hour can create about 0.4 megawatts of power, enough to power 600 homes.[192]Other theoretical applications extend to pretty much anything that engages pressure or action, including minor vibrations. For example, there are projects working to harness the seemingly small-scale energy production; such as texting on a cellphone in a effort to charge the phone while the phone is simply being touched or moved;[193] [194] applications to harvest energy from airflow from airplanes;[195] and even an electric car that uses piezo tech, in part, to charge itself as it travels.[196]
If we think about the enormous mechanical energy wasted by vehicle transport modes and high traffic walking centers such as downtown streets, the potential of that possible regenerated energy is quite substantial. It is this type of systems thinking that is needed in order maintain sustainability, while actively pursuing a global energy abundance.
(4) Material Production/Access
Unlike the prior three sub-sections, which have taken only existing, established methods into consideration with respect to humanity's potential to achieve an abundance[197] of each given focus, this section will necessarily be approached differently.
The problem with creating a basis for an overall material abundance extrapolation in a similar manner, taking into account general raw materials, is that the level of industrial revision needed to embrace the high degree of efficiency sought, is radically different from current traditional practices. In other words, we cannot definitively extrapolate in the same way, using an existing, singular process or genre technology in order to draw such a conclusion about the level of productivity possible on the whole.
This is because the true abundance-generating efficiency mechanism is to be found in the large- scale system orientation, taking into account the synergy present between the sustainability laws inherent to the natural world and the level of efficiency incorporated within the entire societal operation.For example, today there are over one billion automobiles in the world.[198] From a narrow view, the idea of an “abundance” of automobiles would perhaps imply, based on the current property oriented framework, that every human being on the planet should then own a private automobile. Put bluntly, this is the wrong perspective and an outgrowth of a non-synergetic conditioning which is common to the market system's reinforcement of property as value. From the standpoint of efficiency and sustainability it is extremely wasteful to employ 'one automobile per person' due to the fact that a person actually only drives, on average, only about 5% of the time. Otherwise, the automobile sits in parking lots, driveways and the like.
In the city of Los Angeles, California about 1,977,803 automobiles are reported as in use as of 2009.[199] In abstraction, based on this use-time average of 5%, only 98,890 automobiles would actually be needed to meet the transport time needs of the current use demand, assuming a sharing system. In other words, in principle, only 98,890 automobiles would be needed to meet the transport needs of 1,977,803 people.
Furthermore, for the sake of argument, with all other modes of public transport ignored and with the entire population of Los Angeles (3.9 million people)[200] needing to be mobile for 5% a month, only 195,000 automobiles would be needed, in abstraction, to meet the average use time of 3.9 million people.
Likewise, in the United States in 2008, it was recorded that 236.4 million consumer vehicles were being used. With a U.S. population of 313 million, using the 5% use statistic once again, it would take 15.6 million automobiles to meet use demand. That is an 83% decrease in automobile output to meet the needs of all Americans (a 32.4% increase in use or access based on total population), in theory.
Of course, please note that it is well acknowledged here that such an extrapolation is merely for speculation as obviously many other complicating factors come into play in real life that would adjust this equation greatly. The point here is to give the reader a sense of synergy. What should be pointed out is the noted increase in efficiency, where substantially fewer automobiles are needed to meet the transport needs of substantially more people, due to a system-based, synergetic reorientation (in this case, a car “sharing” system).
Again, this is not to dismiss the need for improved urban or public transport, nor does it address the importance of an automobile's design.[201] At the root of this issue is really the subject of “transportation” itself, the reasons why people need such mobility, and how the environment is designed to cater for (or bypass) such needs. This is an enormous, dynamic subject to consider.Also, let it be stated upfront that no matter what real or assumed efficiencies may exist in real life, the goal of seeking post-scarcity, as both a means to relieve human suffering and as a method to adapt to truly efficient and hence sustainable practices, is without debate as a critical point of focus for an expanding society. It could be well argued that only a perverse society would wilfully choose to persevere with a system that knowingly preserves scarcity for profit and establishment preservation when it is intellectually clear that such a condition is no longer needed and hence any such related human suffering resulting is also no longer needed.
As argued prior, the market economy is not just a response to a scarcity-based worldview, it is also a preserver of it. The market structurally requires a high degree of scarcity, as an abundance focused society would eventually mean less labor-for-income, less turnover and less profit on the whole. If society woke up tomorrow to a world where 50% of the human job market was automated and where all food, energy and basic goods could be made available without a price tag due to increased efficiency, needless to say the job market and monetary economy as we know it would collapse.
Value Shift
In order to think properly about the state of our productive capacity to produce life-supporting and standard of living improving goods today, we need to first rationally separate human needs from human wants, with the priority of meeting needs first.
While this distinction may appear like a controversial opinion to many, in a world where now 46% of the total wealth is owned by 1% of the population;[202] in a world where roughly 1 billion do not get basic nutrition;[203] in a world where 1.1 billion people live without clean drinking water and 2.6 billion people lack adequate sanitation;[204] in a world where 100 million people do not have shelter;[205] in a world where 3 billion live on less than $2.50 a day[206] and in a world where 1.2 billion do not even have electricity[207] - perhaps our priorities as a global civilization need to be addressed with respect to the true maintenance of what we might questionably term “civilization”. The truth is, this priority is not a mere poetic gesture; it is a public health requirement.[208]
The process of our physical and psychological evolution has created human needs. Not meeting these virtually empirical needs results in a destabilizing spectrum of physical, mental and social disorders. Human wants, on the other hand, are cultural manifestations that have undergone enormous, subjective change over time, revealing something of an arbitrary nature, in truth. Now, this isn't to say neurotic attachments can't manifest into wants, so much so that they start to take the role of needs, emotionally. However, that is still mostly a cultural condition.
Sadly, again, the market does not separate needs from wants in its basic psychology, which is why scarcity arguments can be extended infinitely in defense of its existence and hence the proposed need to have a competitive, trade-based society, no matter the degree of abundance that can be achieved. This has arguably created a type of neurosis, in fact, where people assume having “infinite wants” and “more and more” is a virtue or even a driver of human progress itself.
Of course, “infinite possibilities” are certainly a reality in many ways, as society cannot predict what technology will materialize many years down the line as influences change and preferences change. However, infinite possibility is about vulnerability and creativity, while still being strategic and intelligent about resource management and use. This is not the same as infinite wants, which sees the human being as insatiable and indiscriminate. Therefore, part of this value shift will be “undoing” the sociological damage done by the psychology inherent to market-based living. A relatively high standard of living can be made available for all human beings assuming, in part, a basic, responsible value shift away from our troubling patterns of wasteful, frivolous acquisition. It is important to restate that the materialism we endure as a society today is a direct response to the economic need to keep money circulating as much as possible. The role of business as we know it is either to service people's existing wants/needs or to invent them in the hope people will conform by showing new demand. A new “widget” put forward by the market is only as viable as the interest of others to purchase it and the use of advertising and marketing has been very influential in creating a culture which sees ownership and acquisition as a sign of social status.[209] This directly assists the need to keep high levels of consumerism in play as GDP and employment are directly related to this pressure. Again, the less interest there is to consume, the less economic growth and hence less demand for jobs. This slows the existing state of a market economy and creates a systemic loss of wellbeing for many.
It can be well-argued that a culture which has decided that acquisition and expansion is the path of progress/success, promoting constant consumption and seemingly infinite “economic growth”, is going to eventually hit the limits of sustainability on a finite planet.[210] In clear terms, this trend is one of disorder.
Social success and progress can only mean, in part, finding balance with the habitat and the other human beings who share the habitat. Sadly, the market system's entire premise contradicts this sustainable value, as the mechanism of economic unfolding does not reward conservation and the reduction of consumption in a direct sense. Put another way, the market is a scarcity-based structural approach that paradoxically seeks increased levels of consumption to operate “efficiently”.
So, an analysis of our material capacity to bring common goods into a “post-scarcity” abundance to exceed the needs of all humans on Earth cannot be discussed without also understanding necessary, sustainability oriented revisions which will substantially reduce our resource-use footprint at the same time.
In short, the new industrial design approach is to deliberately increase the performance, per unit, of how we use our resources, seeking to always move along the route of doing “more with less”. Within this logic, as noted, a series of “pressure” alleviations toward increased sustainability and production simplification/efficiency would occur.
Efficiency Amplifiers
We will call these “efficiency amplifiers” and the following list presents examples of needed structural economic and social changes which assist this optimized efficiency.
1) The pressure for employment for income or “earning a living” is removed.
In the market model, everyone is structurally coerced to engage some form of trade for survival, whether it is trading labor for a wage or creating a product to distribute for profit.[211] This overall pressure, while often touted as an incentive mechanism for social “progress”, actually reduces overall efficiency greatly, as it does creativity and innovation as well. This creates a spectrum of resource and time waste since the interest in income generation and the pressure to produce is often absent existing demand.
The intent and need to do “something” to gain income for survival persists regardless of our modern reality that society may not need everyone to participate in the economic process. In a NLRBE, the idea of everyone being required to produce or sell something is viewed as counterproductive given the trends of ephemeralization and the necessity of now orienting society toward sustainability.
2) Production targeting social classes is removed.
Social stratification, which is a natural consequence of market capitalism, creates the need to produce a spectrum of qualities for a given good genre.[212] This spectrum is not based on utility or having variation of a good as per the personal needs/interests of individuals. Rather, each quality standard is intended to be purchased by (or made “affordable” to) a given income class.
This creates poor quality goods to meet affordability requirements of lower income consumers and hence generates unnecessary waste. In this new strategically sustainable model, no good is created to be “cheap” by relative standards simply because it fits lower class demographic buying patterns. In a NLRBE, there is no lower class demographic.
3) Inefficiency inherent to the competitive practice is removed.
Competition between businesses produces four basic forms of unnecessary inefficiency and hence resulting waste:
(a) Proprietary incompatibility of related goods components (lack of standardization)
(b) Wasteful multiplicity of goods by competing businesses of the same genre
(c) Incentivized good weakness to encourage turnover (planned obsolescence)
(d) Inherent good weakness due to seeking cost efficiency (intrinsic obsolescence)
With respect to (a), in a sustainable economy there would exist a universal standardization of all related genre components wherever possible. In 1801, a man named Eli Whitney was perhaps the first to apply standardization in an impacting way. He produced muskets, and during his time there was no way to interchange the parts of different muskets, even though they were the same overall design. If a musket part broke, the whole gun was useless. Whitney developed tools to do this and after 1801, all parts were full interchangeable.
While most would assume this common sense idea to be prolific across the global industrial community today, the perpetuation of proprietary components by companies that want the consumer to re-purchase any such needed component from them directly, ignoring the possibility of compatibility with other producers, creates not only great waste but also great inconvenience. Similarly, with respect to (b), a wasteful multiplicity of genre goods by competing businesses is generated at all times in the current model. While less obvious to many, the general competitive nature of the market keeps new ideas invisible from competitors during development. Then, a good is produced for purchase that likely has some overall improvement of a given feature. Once that feature is on the market, it is then acknowledged and assessed by competing businesses and the race to continue improvement moves forward, back and forth.
While many argue this “creative warfare” is a driving force of development/innovation of a given product or purpose, the negative and unnecessary consequence is the rapid, wasteful physical obsolescence inherent to each “cycle” of output. In other words, if a notable cell phone feature improvement is obtained by one company, on the heels of a major release by another company that has already started mass production of their phone version without this upgrade, an immediate state of obsolescence is produced, resulting in less optimized products, which could have been avoided if the producers had been working together, as an industrial whole, rather than hiding progress and competing. While it may be argued also that it is only through price and the patterns of consumer interest that the knowing of what is in “demand” or not can be obtained, the truth of the matter is that communication could be made more readily between the design mechanism and the consuming public as well.[213] This bypasses the “price-demand” acceptance/rejection technique that is also wasteful as well since it requires production to occur, in many cases, before the actual demand is fully understood.
As a final point, a globally interlinked, shared data, non-competitive oriented design/production system would also further facilitate the ability to foreshadow component feature improvement over time. This means industry would be able to understand what changes are coming based on progressive trends and design more efficiently, in anticipation of those looming changes.Regarding (c), or what has been termed “planned obsolescence”, the interest to see products fail or be less optimized to motivate repeat purchases of the same basic good would no longer be incentivized. The practice of deliberately designed obsolescence has been a hidden part of the industrial approach since the mid-20th Century when interest in creating economic growth was high.[214]
In a NLRBE, this interest is removed as there is no market incentive to pursue repeat purchases and therefore more optimized efficiency, durability and sustainability strategies can be applied.
Regarding (d) or “intrinsic obsolescence” as it is termed here, all competition for market share seeks to reduce input costs to whatever degree possible in order to remain affordable in the marketplace and hence persuade the consuming public to purchase one version or “brand” of one good over another. This has been gestured in American marketing culture as “producing the best possible goods at the lowest possible prices.”
This inherent inefficiency of seeking to reduce costs creates, as a systemic result, less efficient goods immediately upon production, in the technical sense. Cutting corners in design and production for the sake of preserving money might be considered “economically efficient” in a market context but it is clearly economically inefficient in the real world (physical context) as it creates unnecessary waste over time. This is not to say that there are no limits to production optimization given the fact that true design can only be taken on the whole, with respect to the state of resources at any given time and associated limitations. This is to say that the use of mere profit-oriented “cost efficiency” to limit product quality is a wholly unscientific means for such decision-making.
As expressed in the prior example regarding automobiles and their use-time, in a NLRBE the property system is replaced by an access system which creates a more fluid means of shared use goods which are not needed at all times by a single person. Common examples would be vacation domicile use, transport, seasonal equipment, tools, production equipment and the like.
As an aside, apart from a general overall reduction of production per use time per person, this can assist larger forms of efficiency as far as convenience as well. We can imagine airport or train travel, for example, being redesigned to assist access to various goods locally, so much so that the idea of “packing” a suitcase was no longer needed. This seemingly minor change alone would positively impact queuing, as well as storage in transit, luggage processing machinery, etc. The chain of alleviation is actually quite extensive when given detailed thought.
Clothes, communication tools, recreational items and the like could all be made available at the destination airport or similar facility upon arrival. While this is foreign to many as an idea, especially given the “personalized” oriented nature of our culture, the strife reduced in no longer having to carry large bags and the like could persuade those modern values, given the increased ease. Either way, it comes down to personal choice. In abstraction, a person could literally live without needing to move property around at all, moving around the world at will, without property-oriented inconvenience.
Again, facilitating a means of access, where things can be shared, will allow many more to gain use of goods they otherwise would not in the current model, along with less being produced in proportion. A NLRBE seeks to create access abundance, not a property abundance.
It is also important to note that property is not an empirical concept, only access is. Property is a protectionist contrivance. Access is the reality of the human/social condition. In order for one to truly “own”, say, a computer, one would have had to personally come up with technological ideas that made it work, along with the ideas that comprise the tools of its production. This is literally impossible. There is no such thing as empirical property in reality. There is only access and sharing, no matter what social system is employed.
5) Design-based recycling is mandated and incentivized, maximizing resource reuse.
Contrary to our intuition, there is no such thing as waste in the natural world. Humanity has given very little consideration to the role of material regeneration and how all of our design practices must account for this.
As an aside, the highest state of this recycling will eventually come in the form of nanotechnology. Nanotechnology will eventually facilitate the ability to create goods from the atomic level up and disassemble goods back into raw atoms. Of course, while this approach appears to be on pace for the future, it is not suggested that such nanotechnology is even needed at this time for us to be successfully regenerative or abundant.Today, industrial recycling is more of an afterthought than a focus. Companies continue to do things such as blindly coat materials with certain chemicals that actually distort the properties of that material, making the material less salvageable by current recycling methods. Overall, strategic recycling is a core seed of maintaining abundance. Every landfill on Earth is just a waste of potential.
The law of conservation of mass states that for any system closed to all transfers of matter and energy, the mass of the system must remain constant over time, as system mass cannot change quantity if it is not added or removed. The quantity of mass is "conserved" over time. This natural law implies that mass can neither be created nor destroyed. Human society's use of resources is perhaps best thought of as a process of intelligent rearrangement, rather than of “using” and "discarding".
6) Material use per a given production output is strategically calculated to assure using the most conducive & abundant materials known.
As will be expressed more so in the essay “The Industrial Government”, a new model of evaluation is created which orients materials based on certain efficiency parameters. Two critical ones are material “conduciveness” and a material's overall state of “abundance”.
Conduciveness relates to how appropriate the proposed use is, based on the material's properties. Abundance refers to how much of it is available and hence its state of scarcity. Put together, you weigh the value of conduciveness against the value of how accessible and low impact the material is, as compared to other materials that may be more or less conducive and more or less abundant. In other words, it is a synergistic efficiency comparison that makes sure the materials used are optimized for the purpose.
Probably the best example of this is home or domicile construction. The common use of wood, brick, screws, and the vast array of parts typical of a common house, is comparatively inefficient to more modern, simplified, abundant prefabrication or molded-able materials.
A traditional 2000 square foot home is reported to require about 40 to 50 trees. Compare that with houses that can now be created in prefabrication processes, like mold extrusion, with simple, Earth-friendly polymers, concrete and other easily formable and movable methods. Such new approaches have a very small footprint, as compared to our destruction of global forests for wood. Home construction today is one of the most resource intensive and wasteful industrial mediums in the world today and it doesn't need to be that way.
7) Design conduciveness for labor automation.
The more we conform to the current state of rapid, efficient production processes, the more abundance we can create. Most manufacturing approaches typically divide labor into three categories: human assembly, mechanization and automation. Human assembly means hand- made. Mechanization means using machines to assist the human worker. Automation means no human interaction in the process.Imagine if you needed a chair and there were three designs. The first is elaborate and complex and could only be done by hand at that time. The second is more streamlined where its parts could be made mostly by machines, but would need to be assembled by hand in the end. The third is a chair that is produced by one machine process, fully automated.
This latter chair design type would be the design goal in this new approach. What this would do is reduce the variety of automation machine configurations needed. Imagine, if you will, a robotic-based processing plant that can not only produce cars, it can produce virtually any kind of industrial machine/good comprised of the same basic set of raw materials. This would increase output substantially.
An easy way to understand this trend of simplification is to consider the power of digital software and how one piece of hardware (i.e. computer) can now serve an enormous number of programmable roles. This “dematerialization”, as it could be termed, is best exemplified by the modern cell phone. Due to the vast program applications now available for such “smart phones”, from medical measurements to full musical synthesizers, the functionality of these small, handheld computers can now take on almost countless roles.
Such roles long ago, before the digital age, would have usually required one hardware configuration for each task. Today, any basic operating system can run a dramatically large number of programmed functions, all contained in a small device. This logic applies to the nature of physical machine production as well as it is simply a matter of time before the act of producing a vast array of goods can be accomplished by small, modular mechanical systems, just like a digital operating system can conduct almost countless programmed functions.
8) Serviceable problems resulting from the prior, inefficient economic process are reduced if not eliminated.
This idea is often difficult to fully comprehend, as the chain of causality resulting from one general inefficiency can be vast and complex. For example, the resolution of water scarcity alone has enormous preventative potential for disease. The amount of labor and resources once used for treating those then resolved diseases can find other roles. Energy abundance has the same reality since energy is the driver of all human activity. A clean, reliable, renewable state of absolute energy abundance would have enormous effects on the production and abundance capacity of this future society.Likewise, the pursuit of meeting human needs and the removal of “labor-for-income” occupations, which often have no real technical function, would set in motion a new educational possibility, reinforced by an incentive to pursue personal interests and hence the freedom not to feel pressured away from fields of interest since survival and well-being are already taken care of by the social model itself. It is hard to imagine the explosion of creativity possible when this pressure is removed and society is set free to think clearly.
9) Invigorating the “group mind”, meaning human connection and the sharing of ideas, will bring ever-accelerating progress.
Similar to the prior point, the Internet has become a powerful tool for research and idea expansion. While “open-source” research and development gets a fair amount of attention today, the ability to harness the communicative power of the internet to create a global dialogue about any given technology or idea will facilitate a type of interactive development never before seen, once focused.
The Game Changers
The discussion of advanced technologies which can dramatically transform the unfolding of the future and assist the pursuit of post-scarcity have not been a focus of this essay as it becomes too easy to simply assume the reality of the speculations. A great number of “futurists” have done just this with mixed results and often times it leaves the audience with looming, premature expectations, waiting around for this or that new technology to finally progress. However, to dismiss these potentials is equally as hasty. The truth of the matter is that our capacity to accelerate such change comes down to our focus. Just as the Manhattan Project was able to bring countless scientists together for a single output goal (as violent as it may have been to build the atom bomb), the idea of global network projects to rapidly accelerate new technical possibilities is merely a matter of choice. We can only imagine the progress of any given project if enough minds came together to pursue it at once, in an organized way. This “open-source” world approach alone will likely have limitless possibilities.
Likewise, there is no shortage of transformational or “disruptive” technologies on the horizon that could radically alter the industrial landscape. Artificial intelligence, robotics, biotechnology, 3D printing, infinite computing and nanotechnology are just a few. Each of these developing mediums has vast implications for efficiency increases. It is very difficult to know exactly how they will unfold or, more importantly, how they will find synergy, but we do know the trends of development are increasing exponentially in most cases.
For example, a fusion of 3D printing, nanotechnology, AI and robotics will forever alter the state of manufacturing, so much so that a person could perhaps have a garage size manufacturing system in their home to produce virtually anything they may need. Again, while such futuristic and seemingly “science-fiction” speculations are unneeded to justify our modern, tangible capacity to create abundance, these new and emerging mediums should not be overlooked as they are set to have a great impact, if embraced properly.[215]
In the 19th century, aluminum was more valuable than gold, even though it is technically one of the most abundant elements in the world. However, before the discovery of electrolysis, it was extremely difficult to extract. Once this technical process was discovered, almost overnight the scarcity of the material plummeted. Today, we tend to use aluminum with a throwaway mindset. Such dramatic historical changes are important to keep in mind as the same kind of advancement is occurring across many disciplines, often hidden from most people's comprehension and far beyond their expectations. Likewise, the aforementioned technologies are on pace to dramatically change the world.
Raw Resource Assessment
As noted, assessing the state of natural resources to gauge the degree of total/maximum use capacity as per the human population cannot be done by simply extrapolating around current methods. We need to get both a general sense of current inventory levels of all relevant Earthly, resources and then digest them with respect to the aforementioned efficiency amplifiers which, in effect, radically change the way industrial practice and consumption unfolds. It is also worth noting that modern science has brought a great deal of synthesis into play and the use of polymers, meta-materials and other rapid advancements in chemistry, physics and engineering are accelerating. The end result is that many resources considered problematic, suchas rare Earth metals, are finding replacements via highly abundant means.
It is important to point out that most perspectives on current resource use trends are quite negative by those thinking within the context of the current model.[216] There is no shortage of negative reports and rightly so. We have been abusing and misusing our resources to a vast degree, locked into a life-blind paradigm which has little structural comprehension of its consequences.[217] However, again, this is actually a mismanagement problem, not a quantitative or empirical one.
It is also important to note that it is not how much or how little there is of any one thing in absolute terms. Rather, the qualifier has to do with how we are to achieve the purpose sought. For instance, the available amount of oil in the Earth, as would be needed for its non-energy uses today (since in this model it isn't needed for energy, as noted), is only as relevant as our incapacity/capacity to find other ways to achieve the same goals oil has achieved, but without it.
Another example is lumber. If home construction completely transcended the use of wood frame houses, globally, using Earth-friendly concrete and polymer processes instead, coming from ubiquitous and abundant raw materials, suddenly a once potentially scarce resource becomes exceptionally abundant, relatively speaking.
Moving on, natural resources are best organized initially by dividing them into (a) biotic and (b) abiotic. Biotic resources are derived from the biosphere and are often called “living resources”.[218] Examples of biotic resources are forests, plants, animals, etc. By some definitions, it also includes resources originating from life in the distant past, such as fossil fuels. Abiotic resources are often considered “non-living” resources and include water, soil, minerals and the like.
(a) Overall, the biotic resources of the planet have been suffering greatly due to ever-increasing industrialization. Forest depletion, the loss of biodiversity, loss of fish populations and other issues have brought the sustainability of many such resources into question. In all cases, the problem is not a limited supply of these resources; it is a blatant disregard for any equilibrium with natural regeneration and basic environmental respect. The solution to these declines is to obviously deviate from their rates of use. This can be done by simply substituting other comparable materials for those being harvested at unsustainable rates.
In the essays True Economic Factors and The Industrial Government, this process is described in detail. In short, there is no biotic resource being used today which cannot have its rate of consumption subsided by conscious, strategic adjustment. Wood does not need to be used today for all the current purposes. Not everyone needs to eat fish from the wild ocean as advanced and humane aqua-farming processes now exist. We have already discussed the ability to produce a vegetarian abundance with vertical farming and the move to in-vitro meat can be more healthy and sustainable than livestock methods that are damaging the environment.
With such alleviations, we would see a vast improvement in overall resources, biodiversity, the preservation of life-saving medicine derived from the rainforests and so forth. The other, largely untapped renewables mentioned prior, can also rapidly displace fossil fuels for energy use today. So, the issue is really a matter of intelligent choice.
(b) Abiotic resources have a different, yet similar management reality. We have already addressed our technical ability to circumvent or solve the problem of water scarcity with purification methods and our rapidly depleting topsoil[219] with soilless farming. Overall, the main resources we are left with are the valuable minerals we utilized to build many of the goods we use. These minerals are mostly compounds of Earthly elements and are extracted from rocks from the Earth's crust. Much progress in use-versatility has also been achieved by industry by extracting elements and forming metal alloys. An alloy is a metal mixture made by combining two or more metallic elements, such as the formation of steel.
There are close to 5,000 known minerals[220] and the number of alloys possible is enormous, with many thousands in use today. As far as analysis, the British Geological Survey (BGS) outputs a statistical assessment of world minerals/elements/chemical compounds each year regarding global extraction/production use.[221] 73 are documented in their 2007-2011 report and hence these can be considered the most utilized for global industrial production.[222] Of those, the BGS in turn updates a “risk list” of such materials based on stressed or anticipated stressed supply.
The following chart expresses the medium risk to very high-risk elements, as per their analysis.
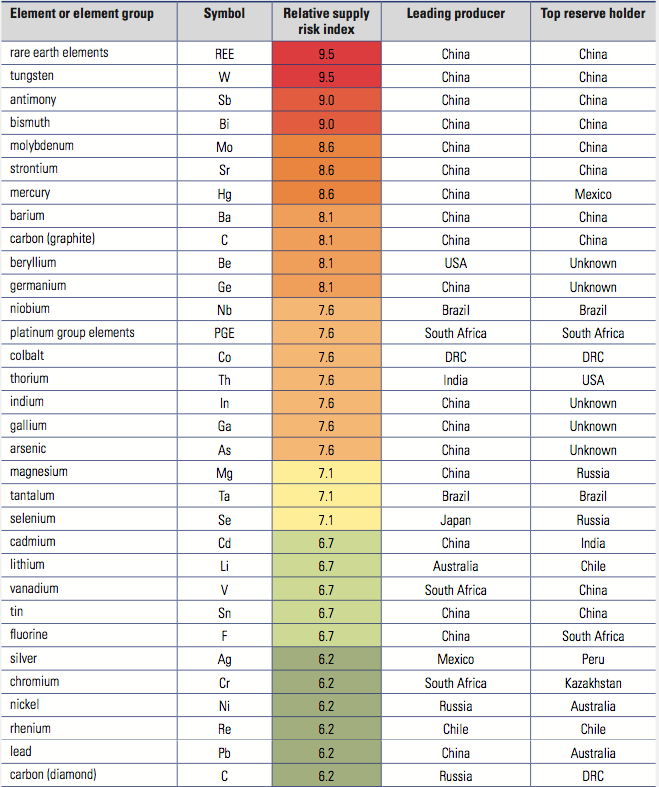
The BGS states “The...list provides a quick and simple indication of the relative risk in 2012 to the supply of...elements or element groups that we need to maintain our economy and lifestyle. The position of an element on this list is determined by a number of factors that might affect availability. These include the natural abundance of elements in the Earth’s crust, the location of current production and reserves, and the political stability of those locations...recycling rates and substitutability of the elements has been considered in the analysis.”[224]
The qualifier of political stability/governance is actually not relevant, empirically. This is a cultural problem. It should be stated upfront that a NLRBE is achieved by global cooperation and the common war patterns, the “resource curse” and disruptions in the supply chain by such contrived, self-preserving pressures common of world powers would no longer be a problem.
Overall, the BGS rightfully concludes that substitutability and recycling are the solutions and the scarcest resources essentially suffer from a lack of recycling and a lack of adequate substitutions being made. Rather than address each material noted, the first one listed, rare Earth metals, will be used as the example by which problem resolution can be considered with all the others.
There are seventeen rare earth metals that are considered the most scare of all elements.
Recycling:The first great failure is that only one percent of all rare Earth minerals are recycled today, according to some estimates.[225] Given their common use in electronics, electronic waste recycling has also been dismal. Based on EPA statistics in the US, in 2009 only 25% of consumer electronics were collected for recycling.[226] Likewise, the goods created that hold most of these valuable materials are also not even intended to be recycled for the most part.[227]
According to an organization called SecondWave Recycling, “for every one million cell phones recycled, we can recover 75 pounds of gold, 772 pounds of silver, and 33,274 pounds of copper...If the United States recycled the 13 million cell phones that are thrown away annually, we could save enough energy to power more than 24,000 homes for a year.”[228]
Substitutions:Perhaps more importantly, it is now possible to manufacture synthetic versions of these metals in the context of their properties out of very common, abundant materials, in a lab.[229] [230] Nanotechnology is proving to be very strong in this approach.[231] Many different industries have been actively working to address the issue in each application, such as now being able to make LED light bulbs without these metals.[232] Overall we see the push to solve this problem ramping up and the fact is, resolution is simply a matter of ingenuity, focus and time.[233]
Industrial reorientation is also important to add to this problem solving equation as a larger tier form of substitutability. While this may not currently apply to rare Earth metals as much at this time, larger scale components in various technologies are changing rapidly. It is a design initiative in engineering to actively focus on component innovation that can bypass such needs. However, given the rate of change for rare Earth metal substitution through synthesis, it appears to be simply a matter of time before this issue is resolved through a combination of strategic use, recycling and synthesis.Beyond that, it cannot be reiterated enough that the great failure of global industry has been not to make proper purpose comparisons when it chooses to use a certain material. In other words, it is not intelligent to use a very rare metal in a generally arbitrary and fleeting product. Since there is no referential database that shows active rates of use, decline and the like, companies make their decisions based merely on cost relationships which have very little value in the sense of strategic use by comparison. While it is true that price can reflect scarcity and difficulty of acquiring a certain mineral or element, such a dire reality arises only as the problem acutely materializes. In other words, no real foresight exists in price and by the time price reflects what was actually an observable technical reality at any time, it is often too late and the scarcity becomes a real problem.In an actively aware resource management system, this would not occur. Not only would such materials be constantly compared to draw assessment as to what is the most appropriate material for a given use, any foreshadowed problem can be seen from a long period away and hence efficiency can be better maximized.[234]
Land
Unlike prior assessments, the issue of land access takes a different consideration. Earth has a finite amount of inhabitable land and hence the method by which humans gain access to and share land over time is the real issue. Needless to say, not every human being can have his or her own private Earth. Likewise, the sickness bred by materialism, wealth and status, which manifests vast and enormous estates by the super rich, fall in the same irrational category – utterly oblivious to sustainability and social balance.Today, the property system creates a static orientation to land access, with people typically acquiring land and staying on it indefinitely. This tendency to “settle” seems compounded by the labor roles and location requirements of most in the world as well. The tradition of commuting to one's job in a city center is still very common and hence one's home needs to be nearby. In a NLRBE, such pressures are greatly alleviated and the idea of traveling the world constantly is a tangible option.Analysts have found that if we needed to fit the world's 7 billion people into a single city, modeled after New York City, all Earthly inhabitants would fit in the US state of Texas.[235] While clearly impractical, this simple statistic reveals the vast degree of variance possible regarding how human beings can organize themselves topographically in a global society. The problem isn't the amount of physical space needed for 7 billion or many times more. The problem is intelligent organization, design and education. That noted, the method of access for a NLRBE is to create an interactive sharing system. The foundation of this idea will be expanded upon greatly in the essay “The Industrial Government”. In short, people are able to travel from destination to destination, enjoying a given location for a period, before likely moving on. Such systems already exist in the current system, where a network of people and domiciles is available for sharing.[236]
Of course, many used to a “home” oriented frame of mind, which has a traditional romanticism, should not be fearful of losing such emotional security. There is no reason why a “permanent” location for a person or family cannot exist, as we find in the world today. In fact, in a society predicated on access abundance, finding and living in a permanent abode would likely be far easier than in a property ownership society.
Yet, statistics prove that today people very much enjoy moving around, exploring and enjoying new places. If it weren't for their labor-for-income job and monetary limitations, it is clear a great deal more traveling would occur by the vast majority. Once such an access system is set in motion, the network of available places to stay and visit would open up and close down in a natural flow, just as hotels work. When a hotel is booked and full for a given day, naturally others seeking to visit that region look elsewhere. As demand ebbs and flows, feedback is used to produce new structures and the like, no different, again, than how it is done today in the vacation market.
The educational and value imperative is the idea of sharing the world. Many today would consider this to be grossly idealized. The idea of freely moving about the planet, staying virtually anywhere, with no obligation to feel the need to return to any central place, seems like a fantasy. Yet, it is very possible. Also, since remote communication is exponentially increasing, engaging in any social/community task or creative interest can occur virtually anywhere as well.
Again, this is a value choice. If a person wishes to keep his family in one place for the rest of their lives, there is more than enough space on the planet (given the Texas statistics noted) to provide for both possibilities, assuming an intelligent revision of city layouts, responsible conservation and an earnest interest to be efficient. Either way, the same access system can be employed to find and settle a certain location, whether it is temporary or permanent.
Oil
In conclusion to this essay, issues surrounding modern society's addiction to the use of oil are important to address. Oil is likely the most industrial resource utilized on the planet today, used most notably for transport. As described prior, between battery technology, improved design and the vast renewable mediums we have today, there is no legitimate technical reason we need gasoline to power automobiles anymore. The handful of currently available electric cars today is also a clear testament to this fact. Airplanes and other extremely large powered machines might still need such oil force currently but the trends show it is simply a matter of time and focus before planes are able to use solar energy[237] coupled with advanced storage means for large scale, heavy weight commercial needs.
Yet, we should always try to think outside of the box when it comes to efficiency and sustainability. In the context of this large-scale, high-energy transport, the question arises: “is there a replacement for plane travel which bypasses such high concentration energy needs?” The answer is yes. Maglev technology is many times faster and uses a fraction of the energy.[238]
So, even if some oil was used for power purposes here and there, such new approaches could reduce its use footprint exponentially, if pursued correctly. In America alone, 70% of the oil used in total goes towards transport in the form of gasoline, diesel and jet fuel.[239] Likewise, if a new condition of peace can be negotiated on planet Earth, with a concentrated pressure to reduce armaments and preparations for war, an extensive oil savings would also occur.
The United States Department of Defense is one of the largest single consumers of energy in the world, responsible for 93% of all US government fuel consumption in 2007.[240] The US military uses more energy than most countries. The military is also one of the greatest polluters in the world.[241] So, working to shut down all military establishments would facilitate a vast increase in this resource's abundance.
Yet, as noted, oil is still polluting in multiple ways so using it as we have for combustion is not environmentally intelligent. The real solution is social revision. While the edifice of human society today has a vast dependence on oil and gas in general, generating all sorts of products from plastics and fertilizers, creative engineers have been slowly challenging this core chemical foundation need for many years.
Plastics, which are ubiquitous in the world today, have been almost exclusively in the territory of petroleum for some time. However, recently Dutch scientists have invented means to replace oil-based plastics by using plant matter.[242] [243] Likewise, an organization called Evocative has been able to use mushrooms to generate fully sustainable materials which can also serve to replace many petroleum uses for insulation and the like.[244]
Overall, a great deal of scientific work is going into substitutes for petroleum and most are plant oils and fats because they have essentially the same base chemical structure as petroleum. So, the real issue again is focus. Today, commercially available, non-petroleum based plastic bottles (“bioplastic”) are becoming much more common[245] so it is clear that the real solution to evolving out of our material petroleum dependence is an issue of intention by the scientific community.
Agriculture is another concern. Fertilizers and pesticides require oil and natural gas and it is well argued that modern civilization, given its rate of food consumption and growth, based on current methods, would not be able to function without these base means. This is likely true. However, that is partly why the prior vertical farming section is so important. Rather than seek to replace these mediums, within the context of traditional agricultural, the solution is to bypass the problem with the new methods.
Overall, if you think of anything oil and hydrocarbons do today, you can either find an establishment-preserving replacement for it (i.e. the plant oil-based plastics which work in most existing industrial contexts) or a completely new approach based on revised methods which bypass the problem altogether (i.e. vertical farming and its little need for such fertilizer). Not to mention, if we remove oil and gas simply from the main combustion purposes, you then free up so much of it that, apart from environmental concerns, the resource becomes that much more abundant, giving even more time to find further solutions to eliminate any and all environmentally unsustainable realities.
TechnoCapitalist Apologetics
At the root of the increased capacity for abundance, as noted prior, is ephemeralization or doing “more with less”. Moore's Law, which is the phenomenon that computer power or chip performance essentially doubles every 18 months, has been found in the modern day to also include any kind of information-based technology.[246] For example, the application of labor automation, which is a combination of robotics and programming, both of which are defined by information in origin, reveals how the means of production itself is becoming an information technology and hence subject to exponential growth as well.
In financial terms, the result of this pattern has been cheaper price values as the efficiency inherent reduces costs to whatever degree allowed. This can be seen in the sharp rise in inexpensive and now ubiquitous technologies, such as cell phones. In absolute abstraction, with all things being equal, assuming society maintained only its current spectrum of use goods, many production trends have the capacity to approach “near zero” value. Given this, the question arises: at what state of such exchange value reduction (price) does value itself become so miniscule as to become moot in and of itself, as an economic factor? Can we expect that potential to occur to such an anticipated high degree in the market system?
The answer is no. The market will never create such large scale, dramatic, post-scarcity implying reductions overall due to its central need for scarcity to keep monetary turnover and hence keep people employed. It is worth noting that many in the modern technology movements still justify the existence of market capitalism, as a means towards “abundance”, by observing this general cost reduction phenomenon. As the argument goes, the unfolding of a given production and its increased demand facilitates “better” production methods and hence more savings by the company means more savings by the consumer. This then makes some goods available, over time, to those who would not have been able to afford them prior. If taken at face value, this observation suggests all goods will approach zero in value overtime, as a given market increases in demand.
The first problem, however, is that this argument simply ignores the vast array of general technical inefficiency which can also, if addressed and solved, create those same reduced costs. In other words, it conflates, erroneously, “market efficiency” and “technical efficiency”. Globalization is a common example. While cheap, primitive, Third World labor might be helpful to bring the cost down of a given product for the American consumer market, the wasted energy, wasted resources and possibly inhumane conditions created/exploited to facilitate that “price advantage” really present deep and caustic inefficiencies, in the broad view.
As an aside, while it is indeed true that certain types of technology, usually computer related, are today widely available for many who otherwise would not be able to afford it, this is a result of scientific ingenuity, not the market. Many traditional economists today make the assertion constantly, that “if it weren't for capitalism...”, etc. The truth is that the market is nothing more than an incentive and delivery system and while the profit motivation may, at times, incorporate high levels of technical advancement which achieve a higher output potential, invigorating this “more with less” phenomenon, this is but one possible outcome amongst many. Many other highly profitable means can be utilized which have zero to negative value in the pursuit of post-scarcity itself.
Perhaps the best way to think about it is as a self-limiting threshold. The profit goal of cost efficiency is to remain “competitive” against other producers, while naturally seeking maximum income to keep employees paid and the structure of the company intact. That is the incentive equation. Obviously, no company wants to make itself obsolete by pursuing a state of extreme efficiency.
Likewise, profit culture is shortsighted by nature. This means that when faced with a decision for cost efficiency, the easiest and most immediate path to realize this change will likely be pursued. That can, again, mean the difference between updating a technical operation to be more efficient in its process of actual production - or simply outsourcing to a developing country which can be paid so little due to existing poverty - if it looks best on paper as far as cost savings. The market sees no difference between the two. Decisions are based merely on the trade value and the end tends to justify the means.
So, as time progresses, the market process may, indeed, continue to make certain high demand goods more accessible to those who couldn't afford them prior. However, that is not evidence that the fruits of a true, post-scarcity oriented society can be obtained on the whole in the same framework. It will only be through a direct revision of society to accept the post-scarcity intent, removing the interest to preserve scarcity, which is common today, that true progress in abundance will be realized. This conclusion is also avoiding the vast array of other large-scale efficiency problems inherent to market capitalism with respect to cultural and environmental sustainability, which have been discussed at length in other essays.
As a final note, the debate over “technological unemployment” has proven to be a powerful revelation in this clash of perceived intentions as well. Capitalist apologists have been hiding behind the idea that while technology does replace human labor, it is also creating it. While this may have been true in the slower moving past, a highly skewed reality has become ever more apparent.[247]
For one, the exponential increases occurring today have proven to be outpacing human educational adaptation greatly. There is no 1:1 job loss to job creation process unfolding in the modern world. Job losses today and job loss possibilities for the future are enormous when the machine applications are reviewed objectively, given the exponential trends. The interesting thing is that this very process of automation is a huge part of creating abundance, even though companies, in the logic of seeking profit, are using it to save money. The result is a complex dichotomy, with fewer human workers and hence less money available as purchasing power.
Of all the symptoms of failure of the capitalist model, this technological unemployment phenomenon just might be the most profound as it really reveals a clash of system functions. Capitalism presupposes that human labor demand will be near constant and all encompassing. Yet, if it is cheaper to employ machines to do human roles, how do we get “spending money” to humans who have now been removed from the labor force due to those very machines? How can the machines continue to produce without the “fuel” of monetary circulation?
In the end, the reduced value argument within the capitalist context simply doesn't work as it assumes a direct balance adjustment between cost reduced price value (saving of money due to mechanization to lower final good price) to meet the ever decreasing purchasing power of the now poorly employed consumers (those jobs removed due to mechanization).The only way this could work is if the profit motive itself was removed, which is essentially impossible if we are to still think within the context of a market economy. The only reason companies employ technology to replace human labor to begin with is to save money and increase their competitive place in the overall economy by some degree. This intention undermines any kind of distribution balance between buying power and cost-savings.
References
- ↑ Source: Two Intellectual Systems: Matter-energy and the Monetary Culture, M. King Hubbert, 1981
- ↑ “Ex Post Facto” is a Latin term that means “done, made, or formulated after the fact”.
- ↑ “Proxy”, defined as “the agency, function, or office of a deputy who acts as a substitute for another” (http://www.merriam-webster.com/dictionary/proxy), is used in this context to describe the market economy as a system that serves to assist industrial and, in many ways, societal operations indirectly. In other words, the system of trade, profit, competition and all other such monetary-market attributes are merely mechanisms that orient behavior in a certain, arguably short-sighted way, absent any kind of larger system perspective.
- ↑ A classic example is the electric car that has been around since the 19th century, showing large-scale efficacy in the 21st century via technological improvements. However, the global shift from the polluting gas engine to the clean electric engine has clearly been stifled by established, profit preserving industries related to the automobile manufacturers and energy providers. It is also worth noting that the very foundation of market efficiency is the mechanism of profit. With this “proxy” mechanism, actions are only taken if they are “profitable”. In real life, we can see a vast array of needed actions to assist social and environmental integrity that have no relevance to the pursuit of profit. In other words, if money cannot be made on a given act, that act isn't deemed of value in the view of the proxy.
- ↑ A classic, large-scale example is the existence of poverty. In order to justify poverty, physically, there must be a clear deficiency in the natural world's capacity to meet the needs of those who have resulted to exist in poverty. If it is found that there is a capacity to eliminate poverty as we know it, hence meaning enough food, shelter, water and other resources and services that can, indeed, be made available without removing those basic, life supporting means from others, then the justification of poverty must find another source. In the current system, this justification is essentially theoretical and determined only by the mechanism of the market itself, implying that if a person is incapable of competing sufficiently to obtain his or her own viable standard of living, then they are hence not deserving of that standard of living. Therefore, the reason poverty exists, as per this example, is the result of the market system and its structure, not real life, physical realities.
- ↑ The main exception to this would be a situation where the assumed system design is simply not understood properly at that time. In other words, if the goal or function of a given system is not fully realized, then naturally that system can only be designed to that level of understanding.
- ↑ Globalization is defined as: “the development of an increasingly integrated global economy marked especially by free trade, free flow of capital, and the tapping of cheaper foreign labor markets”. (http://www.merriam-webster.com/dictionary/globalization)
- ↑ Reference: Globalization: Between Fairness and Exploitation (http://www.pbs.org/wnet/wideangle/episodes/land-of-wandering-souls/globalization-between-fairness-and-exploitation/3073/)
- ↑ Reference: Why globalization is energy intensive and wreaks havoc on oil prices (http://www.csmonitor.com/Environment/Energy-Voices/2013/0228/Why-globalization-is-energy-intensive-and-wreaks-havoc-on-oil-prices)
- ↑ This is a rather subjective example as, ideally, a planned design should be thorough enough to take into account the actual, physical materials and their characteristics, decided upon down to the smallest relevant part. In truth, this only happens to a certain degree, with many components of a given good included as a means to keep a product “cheap” and competitive in the open market. An example would be tires on a car. While general specification of tire grade may be suggested, the use of “cheap” tires could prevail due to an interest to limits costs by the consumer or even the producer.
- ↑ An example of multiplicity is how good production today grades quality based on a targeted demographic. There are deliberately poor goods continuously designed for those with limited purchasing power and there are more optimized goods, which are longer lasting and effective, designed for those who can afford such quality. While native to the logic of the market system's inherent and resulting class stratification, this type of waste is actually unneeded if society had the intention to share design ideas in totality, seeking optimization as strategically as possible. In a NLRBE, this would be the case, removing the market and hence the enormous waste produced by this kind of demographic based multiplicity.
- ↑ See essay True Economic Factors for more on this subject.
- ↑ Here is an example: Fury at Apple's 'rip-off' plan to make ALL iPhone accessories obsolete by changing design of socket (http://www.dailymail.co.uk/sciencetech/article-2162867/Apple-slammed-iPhone-5-charger-Rip-plan-make-ALL-accessories-obsolete.html)
- ↑ A classic historical example of this propensity was what was later termed the “War of Currents”. In short, knowing that his direct current (DC) distribution of electricity was inferior to the emerging alternating current (AC) system put forward by Nikola Tesla, inventor Thomas Edison engaged in an extensive disinformation campaign to suppress AC's popularity, to preserve his financial stake in the existing AC infrastructure. Edison even went so far as going to the United States Congress seeking to outlaw alternating current, along with publicly killing animals by AC electrocution.
- ↑ While the existence of government has served as both a regulator of business matters as well as an active colluder of such matters given its existence as a business in and of itself, there have been very few global actions that have united the industrial system. One example was the Montreal Protocol, which, perhaps for the first time in history, united the world in a basic interest in environmental protection (Ozone Layer Depletion).
- ↑ Industrial design is traditionally defined, as “is the use of both applied art and applied science to improve the aesthetics, ergonomics, functionality, and/or usability of a product.”
- ↑ This issue will be addressed at the end of this essay. Suggested reading: Report Suggests Nearly Half of U.S. Jobs Are Vulnerable to Computerization (http://www.technologyreview.com/view/519241/report-suggests-nearly-half-of-us-jobs-are-vulnerable-to-computerization/)
- ↑ Viewing the world with a “success” and “status” association to material possessions, as is common today, often pollutes considerations of the positive stress reducing, free time enabling, conflict relieving possibilities of labor automation. Does one want to have a life of freedom from monotony, stress and toil or does want to have vast, excess material possessions? This question presents a value system conflict and the term “adaptation” here is to suggest that people may be willing to reduce the complexity of industry a bit by having less material “wants”, in favor of a more “simplified” lifestyle. This isn't to suggest everything we have today could not be available in a NLRBE. It is to say that a worldview which is less materialistic could prevail to make such a transition to a kind automated economy more simple, not to mention be more ecologically sustainable, given the inherent waste.
- ↑ Materials science is an interdisciplinary field involving the properties of matter and its application to various areas of science and engineering. (http://www.sciencedaily.com/articles/m/materials_science.htm)
- ↑ Metallurgy defined: http://www.sciencedaily.com/articles/m/metallurgy.htm
- ↑ Reference: Bronze Age: http://www.thefreedictionary.com/Bronze+Age
- ↑ Source: “New Element 115 Takes a Seat at the Periodic Table”, Time, 2013 (http://science.time.com/2013/08/28/new-element-115-takes-a-seat-at-the-periodic-table/)
- ↑ In 1789, Antoine Lavoisier published a list of 33 chemical elements, grouping them into gases, metals, nonmetals, and earths. In 1870, Russian chemistry professor Dmitri Mendeleev produced the first "periodic table" with about twice as many, with a new element being discovered about ever year on average thereafter.
- ↑ Nanotechnology defined: the science of manipulating materials on an atomic or molecular scale.
- ↑ Reference: Michio Kaku: Can Nanotechnology Create Utopia? (http://betanews.com/2012/11/08/star-trek-replicator-is-closer-to-reality-than-you-think/)
- ↑ “Smart Materials”, as a term, is rather new and ambiguous. A common definition would be: “Materials that can significantly change their mechanical properties (such as shape, stiffness, and viscosity), or their thermal, optical, or electromagnetic properties, in a predictable or controllable manner in response to their environment. Materials that perform sensing and actuating functions, including piezoelectrics, electrostrictors, magnetostrictors, and shape-memory alloys.” However, a “smart” material isn't necessarily a product of nanotechnology, even though nanotechnology can be common as a means of production.
- ↑ “Metamaterials” are defined generally as “exotic composite materials that display properties beyond those available in naturally occurring materials. Instead of constructing materials at the chemical level, as is ordinarily done, these are constructed with two or more materials at the macroscopic level.” One of their defining characteristics is that the electromagnetic response results from combining two or more distinct materials in a specified way that extends the range of electromagnetic patterns because of the fact that they are not found in nature.
- ↑ Actually, this idea was indeed realized but has been applied very rarely as of now. “In the Gym: Clean Energy from Muscle Power”, Time, 2010 (http://content.time.com/time/business/article/0,8599,2032281,00.html)
- ↑ Biomimicry (from bios, meaning life, and mimesis, meaning to imitate) is a design discipline that seeks sustainable solutions by emulating nature’s time-tested patterns and strategies, e.g., a solar cell inspired by a leaf. (http://www.asknature.org/article/view/what_is_biomimicry)
- ↑ Two broad trends worth noting would be ecological & social. Regarding the ecological, it has been noted that most, if not all major life supporting systems are currently in decline. Ref: Data Shows All of Earth’s Systems in Rapid Decline (http://www.ipsnews.net/2011/07/data-shows-all-of-earths-systems-in-rapid-decline/) Regarding social trends - a worthy statistical observation is the apparent general increase in social destabilization or “uprisings” that have been occurring in the past 30 years. A program tracking new reports called (GDELT), shows a startling increase in such events. Ref: Data Map Shows Protests Around the World Increase, With Caveat (http://theglobalobservatory.org/analysis/576-mapping-some-of-the-worlds-unrest-.html)
- ↑ Source: U.N. Raises “Low” Population Projection for 2050 (http://www.worldwatch.org/node/6038)
- ↑ Reference: Yield Trends Are Insufficient to Double Global Crop Production by 2050 (http://www.washingtonpost.com/blogs/wonkblog/wp/2013/07/01/this-unsettling-chart-shows-were-not-growing-enough-food-to-feed-the-world/)
- ↑ Reference: The Coming Global Water Crisis (http://www.theatlantic.com/international/archive/2012/05/the-coming-global-water-crisis/256896/)
- ↑ The hydrocarbon economy and its degree of scarcity has been a controversial subject for many decades. The debate over the current state of supply, globally, has vast disagreement. “Peak Oil”, which has been the categorical context of this debate, spans many decades, with the main conclusion being that this form of energy is finite. Regional depletion, such as the United States peak in the 1970s with respect to conventional oil fields, provides axiomatic evidence that other regions and hence the world itself will eventually peak, with many analysts seeing this occur in the very near future. This, coupled with population growth and increased demand, compounds the problem, if accurate. Ref: (http://www.independent.co.uk/news/science/world-oil-supplies-are-set-to-run-out-faster-than-expected-warn-scientists-6262621.html)
- ↑ The term “post-scarcity” denotes a state that eliminates access scarcity of a given resource or process, usually by means of optimized efficiency regarding production design and strategic use. Needless to say, the idea of achieving total post-scarcity - meaning an infinite, abundant amount of everything for everyone - is rightfully considered an impossibility, even in the most optimistic views. Therefore, this term, as used here, really highlights a point of focus.
- ↑ Monetary economics is rooted in viewing the world from the standpoint of shortages or inefficiencies. It is based around managing scarcity through a system of price and value relationships. The more an item is scare, the more it is valued.
- ↑ The following quotation by historian Jerry Z. Muller embraces this reality well: “Inequality is indeed increasing almost everywhere in the postindustrial capitalist world. But despite what many on the left think, this is not the result of politics, nor is politics likely to reverse it, for the problem is more deeply rooted and intractable than generally recognized. Inequality is an inevitable product of capitalist activity, and expanding equality of opportunity only increases it -- because some individuals and communities are simply better able than others to exploit the opportunities for development and advancement that capitalism affords.” (http://www.foreignaffairs.com/articles/138844/jerry-z-muller/capitalism-and-inequality)
- ↑ Both relative and absolute deprivation, meaning abject poverty and relative socioeconomic status, show a powerful historical correlation to social destabilization and protest. "Occupy Wall St" which sparked a global protest movement in 2011, was based, most specifically, around income inequality. Its most notable slogan, "We are the 99%", denoted the vast wealth differences between 99% of the population and the 1%, coupled with the power abuse inherent because of this imbalance.
- ↑ Carrying capacity defined: http://dictionary.reference.com/browse/carrying+capacity
- ↑ Source: World population monitoring 2001, United Nations (http://www.un.org/esa/population/publications/wpm/wpm2001.pdf)
- ↑ See essay Introduction to Sustainable Thought, section “Ephemeralization”
- ↑ Presently a great fear persists that curtailing or attempting to control the population into more stable reproductive patterns is per se a necessarily violent imposition. Future generations will understand, as a result of access to more information about the planet's carrying capacity, that unchecked "freedom" of reproduction is, in many ways, itself a violent act against the unborn children, and the human race itself, when resource provision and environmental integrity are not factored into the process.
- ↑ Source: Globally almost 870 million chronically undernourished - new hunger report, FAO (http://www.fao.org/news/story/en/item/161819/)
- ↑ Source: Reducing poverty and hunger..., FAO (http://www.fao.org/docrep/003/y6265e/y6265e03.htm)
- ↑ Source: How Many Calories Should I Eat?, MNT (http://www.medicalnewstoday.com/articles/245588.php
- ↑ Source: Feeding the 9 Billion: The tragedy of waste, IME, (http://www.imeche.org/knowledge/themes/environment/global-food)
- ↑ Source: Author: European food waste adds to world hunger, dw.de (http://www.dw.de/author-european-food-waste-adds-to-world-hunger/a-15837215)
- ↑ Reference: Food waste within food supply chains: quantification and potential for change to 2050 (http://www.ncbi.nlm.nih.gov/pmc/articles/PMC2935112/)
- ↑ Topsoil is the top layer of soil that possesses the greatest concentration of organic matter and microorganisms and it is from which plants obtain the overwhelming majority of their nutrients. Today, it is disappearing at an alarming rate primarily due to conventional agricultural practices such as monoculture (the practice of planting one single crop over and over again). Likewise, soil erosion is increasing rapidly with a large number of problematic effects due to inefficient farming practices. Reference: (http://www.ewg.org/losingground/)
- ↑ Ref: http://newearthdaily.com/floating-vertical-farms-could-feed-the-world-with-cheap-plentiful-produce/
- ↑ Dickson Despommier, a professor of environmental health sciences and microbiology at Columbia University, made notable the idea of vertical farming around 1999.
- ↑ Defined: “Cultivation of plants in nutrient solution rather than in soil.” (http://www.thefreedictionary.com/hydroponics)
- ↑ Defined: A technique for growing plants without soil or hydroponic media. The plants are held above a system that constantly mists the roots with nutrient-laden water. Also called aeroculture. (http://www.thefreedictionary.com/aeroponic)
- ↑ Defined: Aquaculture is the symbiotic cultivation of plants and aquatic animals in a re-circulating system. (http://www.growingpower.org/aquaponics.htm)
- ↑ Source: What is Vertical Farming?, Jake Cox, 2009, (http://www.onearth.org/blog/what-is-vertical-farming)
- ↑ Reference: Waste to Energy, alternative-energy-news.info (http://www.alternative-energy-news.info/technology/garbage-energy/)
- ↑ Source: All About: Food and fossil fuels, cnn.com (http://edition.cnn.com/2008/WORLD/asiapcf/03/16/eco.food.miles/)
- ↑ Source: Feeding 9 Billion: Vertical Farming – Singapore (http://www.offgridworld.com/feeding-9-billion-vertical-farming-singapore-video/)
- ↑ Source: 'Mega' Indoor Vertical Farm: Chicago Suburb New Home To Nation's Largest Such Facility (http://www.huffingtonpost.com/2013/03/28/mega-indoor-vertical-farm_n_2971328.html)
- ↑ Aquaponics is a food production system that combines conventional aquaculture (raising aquatic animals such as snails, fish, crayfish or prawns in tanks), with hydroponics (cultivating plants in water) in a symbiotic environment.
- ↑ Source: World’s largest vertical farm is certified organic (http://www.forumforthefuture.org/greenfutures/articles/world%E2%80%99s-largest-vertical-farm-certified-organic)
- ↑ It is worth noting that all major nutritional requirements are technically available in the genre of plant production. Contrary to popular belief, animal products are not required to maintain a high quality human diet. The six major classes of nutrients (carbohydrates, fats, dietary fiber, minerals, proteins, vitamins), omitting water, can all be found in the plant community. (This is not an argument for “vegetarianism”, it is just a fact.)
- ↑ Source: Vertical Farms: From Vision to Reality (http://blogs.ei.columbia.edu/2011/10/13/vertical-farms-from-vision-to-reality/)
- ↑ Source: Country, the City Version: Farms in the Sky Gain New Interest (http://www.nytimes.com/2008/07/15/science/15farm.html?_r=0
- ↑ Generally, in NYC the average length of a north-south block is 1/20th of a mile, or 264 feet. An east-west block is about 1/5th of a mile, or 1,056 feet. So, a square block would be 264 x 1056 = 278,784 square feet, which is equal to 6.4 acres.
- ↑ Source: United States Census Bureau, 2013 (http://www.census.gov/)
- ↑ The city of Los Angeles is 498.3 sq miles that converts to 318,912 acres.
- ↑ 78 Buildings occupying 6.4 acres each equals 499.2 acres used total. 499.2 acres is 0.15% of the total acreage of Los Angeles (318,912)
- ↑ Source: worldometers.info (http://www.worldometers.info/world-population/)
- ↑ 7.2 billion (total population) divided by 50,000 (production capacity of one 30 story vertical farm) equals 144,000 needed structures.
- ↑ 6.4 (acres used per farm) times 144,000 (vertical farms) = 921,600 acres
- ↑ Source: Farming Claims Almost Half Earth's Land, New Maps Show (http://news.nationalgeographic.com/news/2005/12/1209_051209_crops_map.html)
- ↑ 921,600 (acres of land needed to place vertical farms) is 0.006581% of 13,981,811,200. (Total acres of land on earth used for traditional agriculture, both crop and livestock)
- ↑ Source: Farming Claims Almost Half Earth's Land, New Maps Show (http://news.nationalgeographic.com/news/2005/12/1209_051209_crops_map.html)
- ↑ 6.4 acres (which is what has been determined as enough space to feed 50,000 people) goes into 4,408,320,000 (total land used currently for only crop cultivation on earth) 688,800,000 times. 688,800,000, which represent the number of possible facilities where each can produce enough food to feed 50,000 people, translate into an output capacity of 34,440,000,000,000 people that can be fed. (688,800,000 x 50,000 = 34,440,000,000,000)
- ↑ Source: Livestock and climate change (http://cgspace.cgiar.org/bitstream/handle/10568/10601/IssueBrief3.pdf)
- ↑ Source: Livestock a major threat to environment (http://www.fao.org/newsroom/en/News/2006/1000448/index.html)
- ↑ Source: Big-Fish Stocks Fall 90 Percent Since 1950, Study Says (http://news.nationalgeographic.com/news/2003/05/0515_030515_fishdecline.html)
- ↑ Source: World's first lab-grown burger is eaten in London (http://www.bbc.co.uk/news/science-environment-23576143)
- ↑ Freshwater is defined as water that does not contain salt and can be used for human consumption and other processes, such as agriculture. (http://www.merriam-webster.com/dictionary/freshwater)
- ↑ Source: Health through safe drinking water and basic sanitation (http://www.who.int/water_sanitation_health/mdg1/en/)
- ↑ Source: Freshwater Crisis (http://environment.nationalgeographic.com/environment/freshwater/freshwater-crisis/)
- ↑ Source: Water: Use Less-Save More, Jon Clift & Amanda Cuthbert, 2007
- ↑ Source: Compliance and Enforcement National Priority: Clean Water Act, Wet Weather, Combined Sewer Overflows (http://www.epa.gov/compliance/resources/publications/data/planning/priorities/fy2008prioritycwacso.pdf)
- ↑ Source: Water Quality: An Ignored Global Crisis (http://www.businessweek.com/articles/2013-03-21/water-quality-an-ignored-global-crisis)
- ↑ Source: Ashok Gadgil on safe drinking water (http://www.renegademedia.info/books/ashok-gadgil.html)
- ↑ Source: Ultraviolet Disinfection (http://www.nesc.wvu.edu/pdf/dw/publications/ontap/2009_tb/ultraviolet_dwfsom53.pdf)
- ↑ Source: Multi-barrier Disinfection Strategy - New York City (Case Study) (http://www.trojanuv.com/uvresources?resource=403)
- ↑ Source: waterfootprint.org (http://www.waterfootprint.org/?page=cal/waterfootprintcalculator_national)
- ↑ Source: Ibid.
- ↑ Source: Which Nations Consume the Most Water? (http://www.scientificamerican.com/article.cfm?id=water-in-water-out)
- ↑ Source: worldometers.info (http://www.worldometers.info/water/)
- ↑ 9 trillion 972 billion divided by 3 billion
- ↑ Source: Catskill-Delaware Water Ultraviolet Disinfection Facility (http://en.wikipedia.org/wiki/Catskill-Delaware_Water_Ultraviolet_Disinfection_Facility#cite_note-nyc_pr-1)
- ↑ Source: The Worldwide Network of US Military Bases (http://www.globalresearch.ca/the-worldwide-network-of-us-military-bases/5564)
- ↑ Source: Desalination Overview (http://www.idadesal.org/desalination-101/desalination-overview/)
- ↑ Source: Ibid.
- ↑ Source: Flow electrodes may enable large-scale sea water desalination (http://www.rsc.org/chemistryworld/2013/03/sea-water-desalination-capacitive-deionisation)
- ↑ Source: Advancements in Desalination (http://www.weat.org/sanantonio/files/06%20-%20Summer%20Seminar%202013%20-%20Jim%20Lozier%20-%20Adv%20in%20Desal.pdf)
- ↑ Source: Victorian Desalination Plant (http://en.wikipedia.org/wiki/Wonthaggi_desalination_plant)
- ↑ Source: Wonthaggi Desalination Plant (http://www.onlymelbourne.com.au/melbourne_details.php?id=31996#.UljSCWRDp94)
- ↑ 9 trillion, 972 million divided by 150 million
- ↑ Source: The Coastline Paradox (http://grokearth.blogspot.com/2012/04/coastline-paradox.html)
- ↑ Source: World population (http://en.wikipedia.org/wiki/World_population)
- ↑ Source: water.org (http://water.org/water-crisis/water-facts/water/)
- ↑ It is also worth noting that floating, off shore plants are also technically possible. (http://www.bmtdesigntechnology.com.au/design-solutions/floating-desalination-plant/)
- ↑ An advanced technology called the “Slingshot”, invented by Dean Kamen, is a small-scale water purification system that can produce clean water from almost any source, including seawater, by means of vapor compression distillation. It requires no filters, and can even operate using cow dung as fuel.
- ↑ Source: Types of Renewable Energy (http://www.renewableenergyworld.com/rea/tech/home)
- ↑ Source: Fukushima nuke pollution in sea 'was world's worst' (http://phys.org/news/2011-10-fukushima-nuke-pollution-sea-world.html)
- ↑ Source: Renewable Energy (http://www.energyzone.net/aboutenergy/renewable_energy.asp)
- ↑ Source: Energy – The Facts (http://web.worldbank.org/WBSITE/EXTERNAL/TOPICS/EXTENERGY2/0,,contentMDK:22855502~pagePK:210058~piPK:210062~theSitePK:4114200,00.html)
- ↑ Geothermal power in this context isn't to be confused with small scale, geothermal heating/cooling processes that utilizes heat from a few feet below the surface. (i.e. geothermal heat pumps)
- ↑ Source: Geothermal Energy Facts (http://geothermal.marin.org/pwrheat.html#Q1)
- ↑ Source: Back to Basics Video: What Is Geothermal Energy Anyway? (http://www.renewableenergyworld.com/rea/video/view/back-to-basics-video-what-is-geothermal-energy-anyway)
- ↑ Source: Future of Geothermal Energy (http://geothermal.inel.gov/publications/future_of_geothermal_energy.pdf)
- ↑ Source: EIA projects world energy consumption will increase 56% by 2040 (http://www.eia.gov/todayinenergy/detail.cfm?id=12251)
- ↑ Source: Ibid.
- ↑ Source: Geothermal Energy Could Provide All the Energy the World Will Ever Need (http://www.renewableenergyworld.com/rea/news/article/2010/09/geothermal-energy-is-the-solution-for-the-future)
- ↑ Source: Geothermal FAQs (http://www1.eere.energy.gov/geothermal/faqs.html)
- ↑ Source: Geothermal energy emissions of little concern (http://thinkgeoenergy.com/archives/1733)
- ↑ Source: How Does Geothermal Drilling Trigger Earthquakes? (http://www.scientificamerican.com/article.cfm?id=geothermal-drilling-earthquakes&page=2)
- ↑ Source: First Google.Org-funded geothermal mapping reportconfirms vast coast-to-coast clean energy source (http://www.smu.edu/News/2011/geothermal-24oct2011)
- ↑ Source: Plate Tectonics (http://science.nationalgeographic.com/science/earth/the-dynamic-earth/plate-tectonics-article/)
- ↑ Source: First Google.Org-funded geothermal mapping reportconfirms vast coast-to-coast clean energy source (http://www.smu.edu/News/2011/geothermal-24oct2011)
- ↑ Source: geni.org (http://www.geni.org/globalenergy/library/renewable-energy-resources/geothermal.shtml)
- ↑ Source: geo-energy.org (http://geo-energy.org/geo_basics_environment.aspx)
- ↑ 3626 m2 / 404 m2 = 8.975 square meters
- ↑ It is worth noting that geothermal, and, in fact, all other renewables addressed in this text, inherently combine the extraction or harnessing location with the processing and power distribution location. All hydrocarbon sources, on the other hand, require both extraction (i.e. coal) and processing/power production facilities (i.e. refineries/power plants), almost always in separate locations. As per the current example, the mining land required for coal extraction is omitted from the equation. In short, renewables take up substantially less land and have a exceptionally lower environmental impact in this regard.
- ↑ Source: The Carbon Capture Report (http://www.dailyethiopia.com/index.php?aid=1498)
- ↑ 8760 (hrs in a year) x 1000 (MWh) = 8,760,000 Mwh/year.
- ↑ 0.55 zettajoules coverts to about 153 billion MWh
- ↑ 153 billion MWh (total global use) / 8,760,000 MWh (geothermal plant capacity) = 17,465 geothermal plants.
- ↑ Source: worldcoal.org (http://www.worldcoal.org/resources/frequently-asked-questions/)
- ↑ 17,465 / 9 = 1940.5
- ↑ Source: worldcoal.org (http://www.worldcoal.org/coal/uses-of-coal/coal-electricity/)
- ↑ Source: "U.S. National Renewable Energy Laboratory”. February 6th 2007
- ↑ Source: Evaluation of global wind power (http://www.stanford.edu/group/efmh/winds/global_winds.html)
- ↑ Source: The Earth Has Enough Wind Energy Potential To Power All Of Civilization (http://www.businessinsider.com/the-earth-has-enough-wind-energy-potential-to-power-all-of-civilization-2012-9)
- ↑ 8760 (hrs in a year) x 1320 (MW) = 11,563,200 MWh/year.
- ↑ 13,231 x 9000 acres = 119,079,000
- ↑ 119,079,000 acres is 0.32% of 36,794,240,000 acres (total land on Earth)
- ↑ Source: Assessment of Offshore Wind Energy Resources for the United States (http://www.nrel.gov/docs/fy10osti/45889.pdf)
- ↑ Source: Energy in the United States (http://en.wikipedia.org/wiki/Energy_in_the_United_States#cite_note-IEA2012-13)
- ↑ Source: The Earth Has Enough Wind Energy Potential To Power All Of Civilization (http://www.businessinsider.com/the-earth-has-enough-wind-energy-potential-to-power-all-of-civilization-2012-9)
- ↑ Source: thefreedictionary.com (http://encyclopedia2.thefreedictionary.com/solar+energy)
- ↑ Source: World’s biggest solar thermal power plant fires up for first time (http://reneweconomy.com.au/2013/worlds-biggest-solar-thermal-power-plant-fires-first-time-89135)
- ↑ Source: Ivanpah Solar Electric Generating System (http://www.nrel.gov/csp/solarpaces/project_detail.cfm/projectID=62)
- ↑ 153 billion MWh / 1,079,232MWh = 141,767 plants @ 3,500 acres = 496,184,500 acres. 496,184,500 acres is 1.43 % of 36,794,240,000 (total land)
- ↑ Reference: Is Anything Stopping a Truly Massive Build-Out of Desert Solar Power? (http://www.scientificamerican.com/article.cfm?id=challenges-for-desert-solar-power)
- ↑ Source: Solar Plant Generates Power For Six Hours After Sunset (http://www.kcet.org/news/rewire/solar/concentrating-solar/solar-plant-generates-power-for-six-hours-after-sunset.html)
- ↑ Source: Use and Capacity of Global Hydropower Increases (http://www.worldwatch.org/node/9527)
- ↑ Source: Ocean currents can power the world, say scientists (http://www.telegraph.co.uk/earth/energy/renewableenergy/3535012/Ocean-currents-can-power-the-world-say-scientists.html)
- ↑ According to Michael Bernitsas, a professor at the University of Michigan Department of Naval Architecture and Marine Engineering: "...if we could harness 0.1 percent of the energy in the ocean, we could support the energy needs of 15 billion people." [ http://michigantoday.umich.edu/2009/01/story.php?id=7334#.UmB1B2RDp94 ]
- ↑ Source: Our Current Technologies (http://voith.com/en/Voith_Ocean_Current_Technologies(1).pdf)
- ↑ Source: Assessing the Global Wave Energy Potential (http://www.oceanor.no/related/59149/paper_OMAW_2010_20473_final.pdf)
- ↑ Source: Wave farm (http://en.wikipedia.org/wiki/Wave_farm)
- ↑ Source: Tidal Energy (http://ei.lehigh.edu/learners/energy/tidal2.html)
- ↑ Source: lunarenergy.co.uk (http://www.lunarenergy.co.uk/factsFigures.htm)
- ↑ Source: Ocean Energy: Prospects & Potential, Isaacs & Schmitt, with 15% utilization factor & 50% capacity factor. [ http://www.crpm.org/pub/agenda/1384_nathalie_rousseau.pdf ]
- ↑ Renewable Energy Sources and Climate Change Mitigation, Ottmar Edenhofer, 2012, pp505-506
- ↑ Source: Ocean Energy: Position Paper for IPCC (http://ec.europa.eu/research/energy/pdf/gp/gp_events/wrec/wrec_2008_ocean-hydro_alla_weinstein_en.pdf)
- ↑ Source: Ocean currents can power the world, say scientists (http://www.telegraph.co.uk/earth/energy/renewableenergy/3535012/Ocean-currents-can-power-the-world-say-scientists.html)
- ↑ Source: 'Fish Technology' Draws Renewable Energy From Slow Water Currents (http://michigantoday.umich.edu/2009/01/story.php?id=7334#.UmB1B2RDp94)
- ↑ Source: Ocean currents can power the world, say scientists (http://www.telegraph.co.uk/earth/energy/renewableenergy/3535012/Ocean-currents-can-power-the-world-say-scientists.html)
- ↑ Source: Gulf Stream (http://en.wikipedia.org/wiki/Gulf_Stream)
- ↑ Source: Theoretical Assessment of Ocean Current Energy Potential for the Gulf Stream System (http://www.researchgate.net/publication/256495742_Theoretical_Assessment_of_Ocean_Current_Energy_Potential_for_the_Gulf_Stream_System)
- ↑ 8760 (hrs a year) x 13000 MW = 113 million 880 thousands MWhs/yr
- ↑ Source: Energy in the United States (http://en.wikipedia.org/wiki/Energy_in_the_United_States#Consumption)
- ↑ 4.1 billion / 133.880 million
- ↑ Source: Osmotic Power (http://www.sffe.no/?p=2446)
- ↑ Source: First osmosis power plant goes on stream in Norway (http://www.newscientist.com/article/dn18204-first-osmosis-power-plant-goes-on-stream-in-norway.html#.UmCJ-WRDp94)
- ↑ Source: Osmotic Power Play: Energy Recovery Teams with GS Engineering & Construction Corp to Develop Highly Available Renewable Energy Source (http://www.marketwatch.com/story/osmotic-power-play-energy-recovery-teams-with-gs-engineering-construction-corp-to-develop-highly-available-renewable-energy-source-2013-10-15?reflink=MW_news_stmp)
- ↑ Renewable Energy Sources and Climate Change Mitigation, Ottmar Edenhofer, 2012, p507
- ↑ Source: Ocean Thermal Power Will Debut off China's Coast (http://www.scientificamerican.com/article.cfm?id=ocean-thermal-power-will-debut-off-chinas-coast)
- ↑ Source: 100-mw OTEC project planned for West Oahu (http://www.bizjournals.com/pacific/print-edition/2012/10/05/100-mw-otec-project-planned-for-west.html?page=all)
- ↑ Source: Ocean Thermal Energy Conversion Could Power All Of Hawaii's Big Island (http://www.huffingtonpost.com/2013/09/16/ocean-thermal-energy-conversion-hawaii_n_3937367.html)
- ↑ Source: Renewable Energy Essentials: Hydropower (http://www.iea.org/publications/freepublications/publication/Hydropower_Essentials.pdf)
- ↑ Source: eia.gov (http://www.eia.gov/tools/faqs/faq.cfm?id=97&t=3)
- ↑ Source: Total Number of U.S. Households (http://www.statisticbrain.com/u-s-household-statistics/)
- ↑ Source: List of countries by electricity consumption (http://en.wikipedia.org/wiki/List_of_countries_by_electricity_consumption)
- ↑ While the current state of CPV solar efficiency, as of 2013 is 44.7% [ http://www.soitec.com/en/news/press-releases/world-record-solar-cell-1373/ ], with consumer available products averaging only about 18% [ http://sroeco.com/solar/most-efficient-solar-panels ], more advance methods are suggesting upwards of 80%. [ http://www.extremetech.com/extreme/168811-new-nano-material-could-boost-solar-panel-efficiency-as-high-as-80 ] Likewise, battery storage for household use has been advancing via emerging graphene super capacitors [ http://www.gizmag.com/graphene-based-supercapacitor/28579/ ] which can charge faster, last many times longer than conventional batteries and are less polluting and take up less space. Another advancing battery technology is termed the LMB, as developed by Donald Sadoway and MIT.
- ↑ It is worth mentioning once again that all business establishments perpetuate themselves mostly by the markets they have created prior. A new invention that can interfere with the existing income infrastructure of a given business is often subject to influences that slow or even suppress that income interfering technology. While many see this kind of behavior as a form of “corruption”, the truth of the matter is that the very mechanism of bringing a given good to the market is subject to imposed financial limitations which achieve the same goal. For example, if the new good cannot be considered profitable during the course of its development, regardless of its true merit, it will be hindered. The extremely slow pace of renewable energies on the whole, even though the principle of most means has been understood for hundreds of years, is a direct result of monetary investment or lack thereof and the more efficient a technology, the less profit with be realized in the long-term.
- ↑ Source: Off-Grid System Cost Guide (http://www.wholesalesolar.com/StartHere/OFFGRIDBallparkCost.html)
- ↑ Example: Saving Energy in West Michigan Honeywell Wind Turbine (http://www.freepowerwindturbines.com/honeywell_wind_turbine.html)
- ↑ Reference: Geothermal Heat Pumps (http://energy.gov/energysaver/articles/geothermal-heat-pumps)
- ↑ A design approach called “passive solar” is a good design example. [ http://en.wikipedia.org/wiki/Passive_solar_building_design ]
- ↑ Reference: thefreedictionary.com (http://www.thefreedictionary.com/piezoelectric)
- ↑ Reference: Energy-Generating Floors to Power Tokyo Subways (http://inhabitat.com/tokyo-subway-stations-get-piezoelectric-floors/)
- ↑ Reference: Six Sidewalks That Work While You Walk (http://www.treehugger.com/clean-technology/six-sidewalks-that-work-while-you-walk.html)
- ↑ Reference: Israel Highway Equipped With Pilot Piezoelectric Generator System (http://www.greenoptimistic.com/2009/10/06/israel-piezoelectric-highway/#.UmHNTmRDp94)
- ↑ Reference: New Piezoelectric Railways Harvest Energy From Passing Trains (http://inhabitat.com/new-piezoelectric-railways-harvest-energy-from-passing-trains/)
- ↑ Reference: Under Highway Piezoelectric “Generators” Could Provide Power to Propel Electric Cars (http://www.greenprophet.com/2010/09/piezoelectric-generators-electric-cars/)
- ↑ Reference: Piezoelectric kinetic energy harvester for mobile phones (http://www.energyharvestingjournal.com/articles/piezoelectric-kinetic-energy-harvester-for-mobile-phones-00002142.asp?sessionid=1)
- ↑ Reference: Cisco's Laura Ipsen: Smart grid success requires infotech, energy tech savvy (http://www.smartplanet.com/blog/science-scope/charge-your-phone-by-typing-on-it/8797)
- ↑ Reference: Harvesting energy from vehicle air flow using piezoelectrics (http://www.gizmag.com/harnessing-vehicle-air-flow-energy/13414/)
- ↑ Reference: P-Eco Electric Concept Vehicle Powered by Piezoelectricity (http://psipunk.com/p-eco-electric-concept-vehicle-powered-by-piezoelectricity/)
- ↑ As will be explained more so in this section, the abundance state sought has to do with use-time and access, not outright property. An access society is very different from a propertied one in many profound ways, especially when it comes to sustainability, values and human behavior itself. Food, energy and water already assume an access state since such “items” are perishable or part of an continuum that separates it from physical “good” ownership as we traditionally think of it.
- ↑ Source: Number Of Cars Worldwide Surpasses 1 Billion; Can The World Handle This Many Wheels? (http://www.huffingtonpost.ca/2011/08/23/car-population_n_934291.html)
- ↑ Source: The City of Los Angeles Transportation Profile (http://www.gu.se/digitalAssets/1344/1344071_city-of-la-transportation-profile.pdf)
- ↑ Source: United States Census Bureau, 2013 (http://www.census.gov/)
- ↑ Many other influences and outcomes can arise to the effect of increasing efficiency in such a context. For example, with about 1.2 million deaths occurring annually from automobile accidents, many design initiatives to assist future safety could dramatically alter that reality. The use of sensor rigged, driverless cars, which are now a reality, could end such deaths immediately. Given about 50 million auto accidents worldwide each year, the result is not only saved lives, but saved medical expenses, insurance claims, lawsuits, data entry, resources, time, the toil of stress and grief that result from injury or death, and a massive array of other alleviations.
- ↑ Source: 46 per cent global wealth owned by richest 1 per cent: Credit Suisse (http://profit.ndtv.com/news/economy/article-46-per-cent-global-wealth-owned-by-richest-1-per-cent-credit-suisse-369109)
- ↑ Source: U.N.: One billion worldwide face starvation (http://www.cnn.com/2009/WORLD/europe/11/15/un.hunger/)
- ↑ Source: Water Crisis: Towards a way to impove the situation (http://www.worldwatercouncil.org/library/archives/water-crisis/)
- ↑ Source: An estimated 100 million people worldwide are homeless. Source: United Nations Commission on Human Rights, 2005. (http://www.homelessworldcup.org/content/homelessness-statistics)
- ↑ Source: Causes of Poverty (http://www.globalissues.org/issue/2/causes-of-poverty)
- ↑ Source: Here’s why 1.2 billion people still don’t have access to electricity (http://www.washingtonpost.com/blogs/wonkblog/wp/2013/05/29/heres-why-1-2-billion-people-still-dont-have-access-to-electricity/)
- ↑ Social stability is directly correlated to public health. For example, social inequality can, as it often does, manifest as violent behavior, civil protest and even war, just as poor sanitation and poverty stricken areas can bring disease that could spread to areas which do have good sanitation, yet cause infection (perhaps even an epidemic). Easing economic stress and improving public health is a global imperative for true safety.
- ↑ See the essay Value System Disorder.
- ↑ Source: Ongoing global biodiversity loss and the need to move beyond protected areas: a review of the technical and practical shortcomings of protected areas on land and sea (http://www.int-res.com/articles/theme/m434p251.pdf)
- ↑ The only two exceptions to this are either to go live outside of the civilization itself which, due to property laws, is essentially impossible, or to obtain enough wealth to begin with via the market or inheritance where there is no need for further trade for survival. The latter, of course, is not open to all in a market economy of any kind.
- ↑ All goods created assume a class relationship. The spectrum could range from the type of extreme poor production found at a “99 Cent” store where one could purchase a plastic watch which has little integrity, vs an extreme luxury item which can only be afforded by the most wealthy in the world. Thorstein Veblen inspired the term “Veblen Good” due to his observation of prestige generated from extremely high priced goods, which transcend utility. http://www.investopedia.com/terms/v/veblen-good.asp
- ↑ This will be discussed in the essay The Industrial Government
- ↑ Reference: Ending the Depression Through Planned Obsolescence, Bernard London, 1932
- ↑ With respect to such materials, emerging nanotechnology, such as carbon nanotubes (CNT), which can be arranged to create what has been termed “Buckypaper”, is an example of tremendous potential. “Buckypaper” is a macroscopic aggregate of carbon nanotubes that owes its name to R. Buckminster Fuller. Paper-thin and lightweight, it is one- tenth the weight yet potentially 500 times stronger than steel when its sheets are stacked to form a composite. It can also conduct electricity like copper or silicon. This synthetic material, made out of abundant carbon, could be at the foundation of a new, scarcity transcending synthetic materials revolution.
- ↑ Reference: Two-thirds of world's resources 'used up' (http://www.theguardian.com/science/2005/mar/30/environment.research)
- ↑ In a 2011 study entitled “Ongoing global biodiversity loss and the need to move beyond protected areas: a review of the technical and practical shortcomings of protected areas on land and sea” the following conclusion was made: “In a business-as-usual scenario, our demands on planet Earth could mount to the productivity of 27 planets by 2050.” There is no shortage of other negative resource “overshoot” statistics in peer review as well.
- ↑ Source: biology-online.org (http://www.biology-online.org/dictionary/Biotic_resource)
- ↑ Source: The lowdown on topsoil: It's disappearing (http://www.seattlepi.com/national/article/The-lowdown-on-topsoil-It-s-disappearing-1262214.php)
- ↑ Source: Mineral (http://en.wikipedia.org/wiki/Mineral)
- ↑ Source: World mineral statistics (http://www.bgs.ac.uk/mineralsuk/statistics/worldStatistics.html)
- ↑ Source: World Mineral Production 2007-2011 (http://www.bgs.ac.uk/downloads/start.cfm?id=2701)
- ↑
Source: Risk list 2012: An updated supply risk index for chemical elements or element groups which are of economic value (http://www.bgs.ac.uk/mineralsuk/statistics/risklist.html) - ↑ Ibid.
- ↑ Source: Rare Earth Recycling (http://www.molycorp.com/technology/rare-earth-recycling/)
- ↑ Source: Statistics on the Management of Used and End-of-Life Electronics (http://www.epa.gov/osw/conserve/materials/ecycling/manage.htm)
- ↑ Source: Dirty, dangerous and destructive – the elements of a technology boom (http://www.theguardian.com/commentisfree/2011/sep/26/rare-earth-metals-technology-boom)
- ↑ Source: Why Recycle Cell Phones? Why not just throw it away? (http://secondwaverecycling.com/why-recycle-cell-phones-why-not-just-throw-it-away/)
- ↑ Source: Nanosys: We Can Replace Some Rare Earth Metals (http://www.fastcompany.com/1705030/nanosys-we-can-replace-some-rare-earth-metals)
- ↑ Source: Thin Film Solar Cells Using Earth-Abundant Materials (http://www.intechopen.com/download/get/type/pdfs/id/39155)
- ↑ Source: New Nano Material Could Replace Rare Earth Minerals In Solar Cells and OLEDs (http://inhabitat.com/new-nano-material-could-replace-rare-earth-minerals-in-solar-cells-and-oleds/)
- ↑ Source: New material could lead to cheaper, more eco-friendly LEDs (http://www.gizmag.com/silicon-led-rare-earth-element-alternative/27933/)
- ↑ Source: Rare-earth mineral substitutes could defeat Chinese stranglehold (http://www.wired.co.uk/news/archive/2013-07/31/race-for-rare-earth-minerals)
- ↑ This is expanded upon in the essay The Industrial Government.
- ↑ Source: If the world’s population lived in one city… (http://persquaremile.com/2011/01/18/if-the-worlds-population-lived-in-one-city/)
- ↑ Reference: airbnb.com (https://www.airbnb.com/)
- ↑ Reference: Solar-Powered Airplane Completes First Leg Of U.S. Flight (http://www.npr.org/blogs/thetwo-way/2013/05/05/181407952/solar-powered-airplane-completes-first-leg-of-u-s-flight)
- ↑ Reference: New York to Beijing in two hours without leaving the ground? (http://www.gizmag.com/et3-vacuum-maglev-train/21833/)
- ↑ Reference: Petroleum (http://www.instituteforenergyresearch.org/energy-overview/petroleum-oil/)
- ↑ Source: Colonel Gregory J. Lengyel, USAF, The Brookings Institution, Department of Defense Energy Strategy, August 2007.
- ↑ Reference: The Elephant in the Room: The U.S. Military is One of the World’s Largest Sources of C02 (http://www.washingtonsblog.com/2009/12/removing-war-from-global-warming.html)
- ↑ Source: Who Needs Oil When Scientists Can Make Plastic From Plants? (http://gizmodo.com/5885953/who-needs-oil-when-scientists-can-make-plastic-from-plants)
- ↑ Source: Supported Iron Nanoparticles as Catalysts for Sustainable Production of Lower Olefins (http://www.sciencemag.org/content/335/6070/835.abstract)
- ↑ Source: Mushroom Materials (http://www.ecovativedesign.com/mushroom-materials/)
- ↑ Reference: Coke, Ford join forces to juice supply of plant-based plastic (http://www.greenbiz.com/blog/2012/06/14/coca-cola-nike-ford-join-forces-juice-supply-plant-based-plastic)
- ↑ Reference: Big Idea: Technology Grows Exponentially (http://bigthink.com/think-tank/big-idea-technology-grows-exponentially)
- ↑ Reference: Coming to an office near you (http://www.economist.com/news/leaders/21594298-effect-todays-technology-tomorrows-jobs-will-be-immenseand-no-country-ready)
Source
- This essay belong to Zeitgeist Movement Defined: Realizing a New Train of Thought